ADVERTISEMENT
Programming of the Individual Phases of the Defibrillation Waveform to Achieve an Adequate Defibrillation Safety Margin: Utilization of a Surrogate Cardiac Membrane Time Constant
Achieving an adequate defibrillation safety margin can sometimes be troublesome. Today’s implantable defibrillators allow for the programming of vector and polarity to overcome some of these issues. Some devices also allow the programming of the pulse width of each phase of the defibrillation waveform. This case illustrates the value of proper programming of the first and second phase of the waveform as an aid in achieving adequate safety margins. The measurement of a surrogate cardiac membrane time constant can be utilized in the selection of the proper pulse widths.
Case Study
A 53-year-old female underwent implantation of a biventricular defibrillator for worsening heart failure symptoms. She has a history of non-ischemic dilated cardiomyopathy with an ejection fraction of 35% via echocardiography, ventricular tachycardia, hypertension and dyslipidemia. Twelve-lead ECG revealed normal sinus rhythm with a left bundle branch block and a QRS duration of 130 ms. Her medications include furosemide, lisinopril, carvedilol, spironolactone and simvastatin.
The patient underwent surgical placement of a biventricular defibrillator system for treatment of her heart failure and ventricular tachycardia. A St. Jude Medical model 1888TC lead (Sylmar, CA) was placed in the right atrial appendage. A dual coil defibrillation lead (model 7120, St. Jude Medical) was placed in the right ventricular apex and a St. Jude Medical model 1158 lead was placed in a posterolateral branch of the coronary venous system. The leads were attached to a biventricular defibrillator (model 3207-36, St. Jude Medical) that inserted into a subcutaneous, left clavicular pocket.
Pacing and sensing thresholds were all well within an acceptable range. High voltage lead impedance was measured at 42Ω. A surrogate cardiac membrane time constant of 33 ms was measured via the implanted device. (Figure 1) According to our assumptions, this measurement indicates that patient’s cardiac time constant (tau, τ) falls into the fast, or 2.5 ms range. Programming of the first and second phases (P1/P2) of the biphasic defibrillation waveform was initially programmed to correspond to a typical time constant (3.5 ms). This yielded a P1/P2 of 4/3.5 ms. The defibrillator was programmed to deliver 500V (~12J) as the first rescue shock, 650V (~21J) as the second rescue shock, and a full output shock of 830V (~35J) for all subsequent shocks.
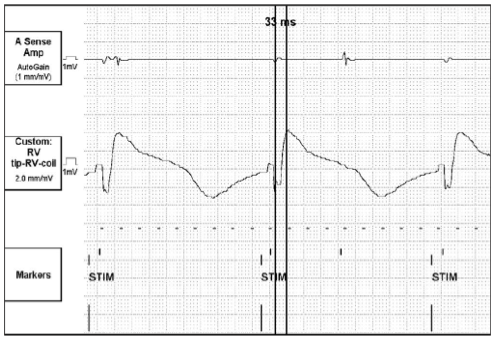
Defibrillation safety margin testing was carried out and ventricular fibrillation (VF) was induced via DC Fibber© at a cycle length of 180 ms. The first rescue shock of 500V (12J) failed to convert the arrhythmia. A second rescue shock of 650V (21.9J) converted the patient to sinus rhythm. (Figure 2) Although 650V resulted in an acceptable defibrillation safety margin of 180V (~13J), P1/P2 was reprogrammed to coincide with a fast time constant, given the evoked response rise time constant of 33 ms. This resulted in P1/P2 values of 3/2 ms. The evoked response rise time is about 20 times the time constant. This allows us to make a surrogate measurement which, in turn, allows an estimate of the actual membrane time constant.
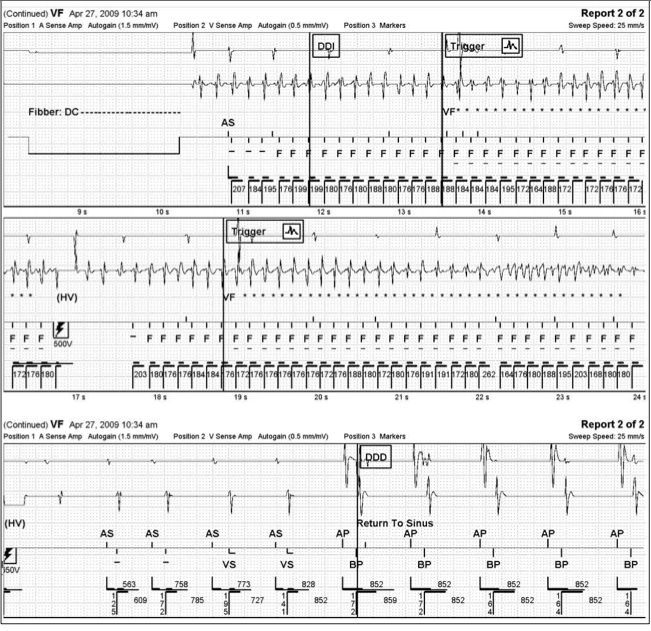
After a recovery period of five minutes, VF was again induced via DC Fibber© at a cycle length of 180 ms. A first rescue shock of 500V (11.4J) successfully converted the patient to sinus rhythm. (Figure 3) Reprogramming of the device in this manner achieved a safety margin of 330V (~23J).
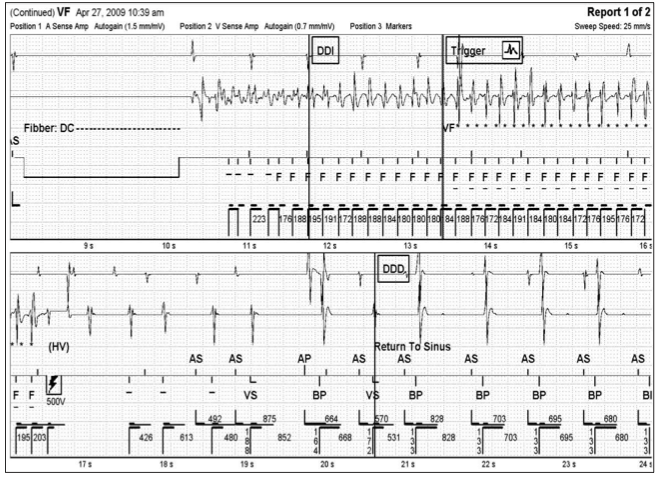
Discussion
It is important when attempting to achieve an adequate defibrillation safety margin to match the time constant of the system (device, leads, patient interface) to that of the patient’s own cardiac tissue time constant (τ).1 The optimal pulse width values depend on the high voltage impedance and the capacitance of the device. Direct measurement of tau is not possible outside of scientific laboratories. Mowrey et al was able to measure cardiac time constant during defibrillation in rabbit hearts with optical mapping techniques.2 This is not practical in the typical electrophysiology lab. Time constants range from 2.5–4.5 ms in humans.1 By measuring the duration of an evoked potential using a vector from the tip of the RV lead to RV shocking coil, one can determine a surrogate cardiac time constant. This measurement is a summation of cell-to-cell conduction time. The faster the cell-to-cell conduction time, the faster then is the cardiac tissue time constant. Slower cell-to-cell conduction would indicate a slower time constant. According to our assumptions, a fast time constant (2.5 ms) corresponds to surrogate tau measurements of less than 40 ms. A slow time constant (4.5 ms) corresponds with surrogate tau measurements greater than 75 ms and a typical time constant (3.5 ms) corresponds with surrogate tau measurements between 40 and 75 ms.
Truncating phase 1 is ideally done at a time when a critical mass of the cardiac myocytes has achieved their peak membrane response. This allows for the capture and refractory-period extension of already depolarized cells.3 Phase 2 returns this charge to baseline and removes any excess charge in the vicinity of the virtual electrode, effectively “burping” the non-captured cells of residual charge — and also discharging virtual electrodes — to reduce the chances of re-inducing the arrhythmia4 and healing electroporated cells.
By programming the device to shorter pulse widths, which are associated with a faster cardiac membrane time constant, this resulted in a shock energy of 500V (11.4J), successfully converting VF to sinus rhythm. This shock energy level failed with pulse widths that corresponded to a typical cardiac membrane time constant. In this particular patient, the cardiac time constant fell into the fast (2.5 ms)5 category. Programming of pulse widths to correspond to a typical time constant (3.5 ms) was shown to be inferior to the predicted fast time constant as 500V failed to convert the arrhythmia to sinus rhythm.7 Figure 4 displays the programming for fast, typical and slow cardiac membrane time constants. These pulse widths are calculated based on high voltage impedance and the capacitance of the ICD. Keane et al was able to show a striking improvement in defibrillation safety margin when using fixed pulse width defibrillation waveforms when compared to 65%/65% tilt waveforms.8 This case demonstrates the utility of indirectly estimating the membrane time constant in order to reduce the DFT.
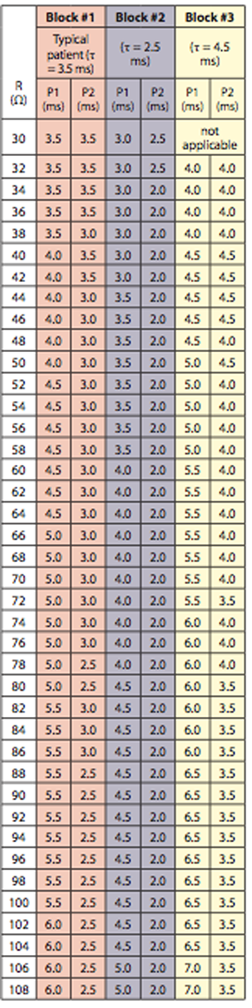
Editor’s Note: This article underwent peer review by one or more members of EP Lab Digest®’s editorial board.
References
- Kroll MW, Swerdlow CD. Optimizing defibrillation waveforms for ICDs. J Interv Card Electrophysiol 2007;18:247–263.
- Mowrey K, Efimov I, Cheng Y. Membrane time constant during defibrillation strength shocks in intact heart: Effect of Na2 and Ca2+ channel blockers. J Cardiovasc Electrophysiol 2009;20:85–92.
- Dillon SM. Synchronized repolarization after defibrillation shocks: A possible component of the defibrillation process demonstrated by optical recordings in rabbit hearts. Circulation 1992;85:1865–1878.
- Kroll MW, Schwab JO. Achieving low defibrillation thresholds at implant: Pharmacologic influences, RV coil polarity and position, SVC coil usage and position, pulse width settings, and the azygous vein. Fundam Clin Pharmacol 2010;24:561–573.
- Denman RA, Umesan C, Martin PT, et al. Benefit of millisecond waveform durations for patients with high defibrillation thresholds. Heart Rhythm 2006;3:536–541.
- Kroll MW. A minimal model of the single capacitor biphasic waveform. Pacing Clin Electrophysiol 1994;17:1782-1792.
- Val-Mejias JE, Doshi SK, Pittaro M, Reeves R, Lee K. In search for perfection: a specific combination of SVC coil location and choice of time-constant provides lower DFTs. Europace Journal 2009, 11(Supplement 2), Abstract 735.
- Keane D, Aweh N, Hynes B, et al. Achieving sufficient safety margins with fixed duration waveforms and the use of multiple time constants. Pacing Clin Electrophysiol 2007;30:596-602.