ADVERTISEMENT
Update on Management of Ventricular Tachycardia in Structural Heart Disease: A Case-Based Review
© 2023 HMP Global. All Rights Reserved.
Any views and opinions expressed are those of the author(s) and/or participants and do not necessarily reflect the views, policy, or position of EP Lab Digest or HMP Global, their employees, and affiliates.
EP LAB DIGEST. 2023;23(6):1,5-8.
Case Presentation
The patient is a 77-year-old man with ischemic cardiomyopathy due to a large inferolateral myocardial infarction due to occluded right coronary stent and akinesis with thinning of the inferior wall. He developed sustained monomorphic ventricular tachycardia (VT) requiring appropriate implantable cardioverter-defibrillator (ICD) therapies. Previously, he experienced inappropriate shocks for both rapid atrial flutter and atrial fibrillation (AF), leading to ablation of both atrial arrhythmias without further inappropriate shocks. He was placed on amiodarone; mexiletine was sequentially added. Due to recurrent visual side effects and ongoing VT episodes despite medical therapy, the patient underwent radiofrequency (RF) ablation for VT.
This initial procedure targeted inferolateral substrate and VT utilizing the inferolateral scar. Despite noninducibility after the first VT procedure, the patient experienced recurrence of slow, sustained, hemodynamically tolerated VT (Figure 1) at 1 year postprocedure. He later underwent repeat VT ablation.
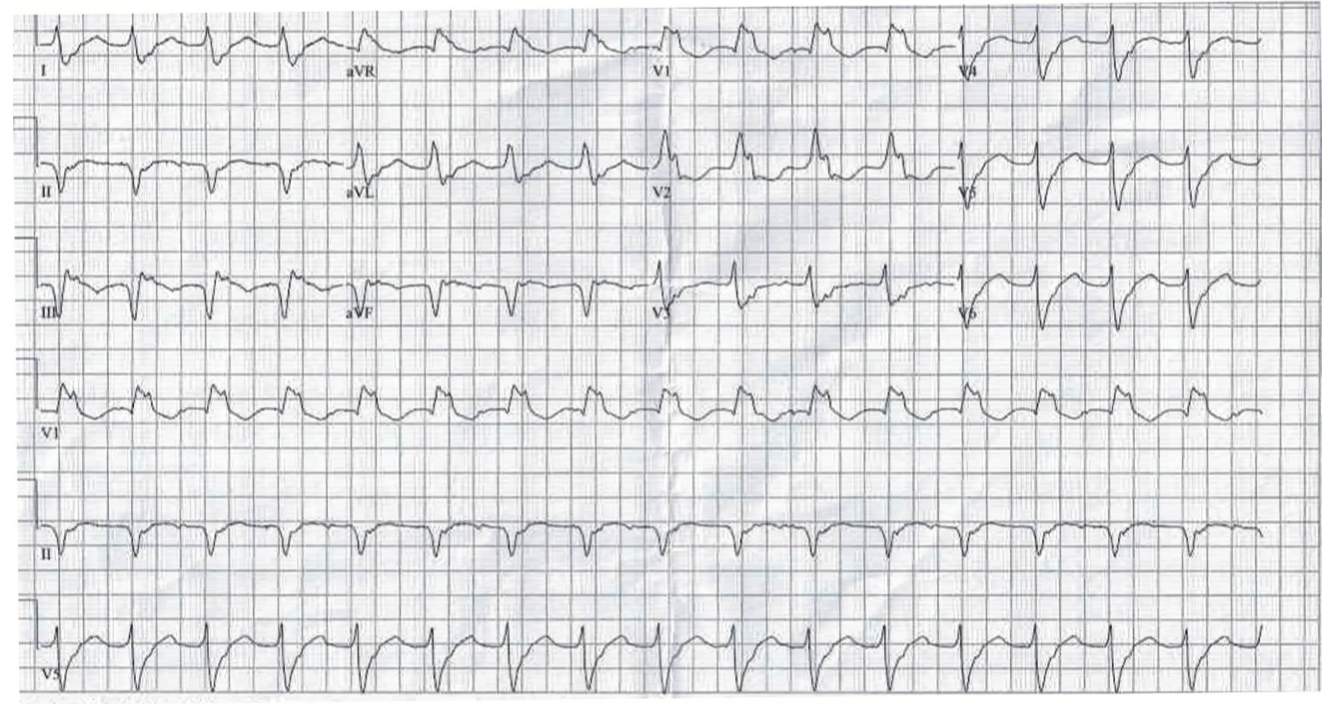
Video 1
Video 1. Activation of inducible VT with a LBLS morphology of 400 milliseconds
Figure 2 demonstrates an electroanatomic voltage map using a high-density multipolar catheter (EnSite X EP System and Advisor HD Grid Mapping Catheter, Sensor Enabled, Abbott), with extensive inferior, inferoseptal, and inferolateral scar extending from the mitral valve annulus to mid-apical left ventricle (LV). Additional VT morphology was inducible during the procedure (Figure 3) with a more apical exit that was not hemodynamically tolerated. Sinus rhythm (SR) mapping demonstrated an area of isochronal late activation in the basal inferior wall (Figure 4A), which correlated with a critical isthmus for the inducible VT (Figure 4B and Video). Extensive substrate ablation targeting areas of isochronal crowding and critical isthmus of the VT circuit rendered the patient noninducible for ventricular arrhythmias (VAs) (Figure 5). At 6-month follow-up, the patient has remained arrhythmia free and off antiarrhythmic medication.
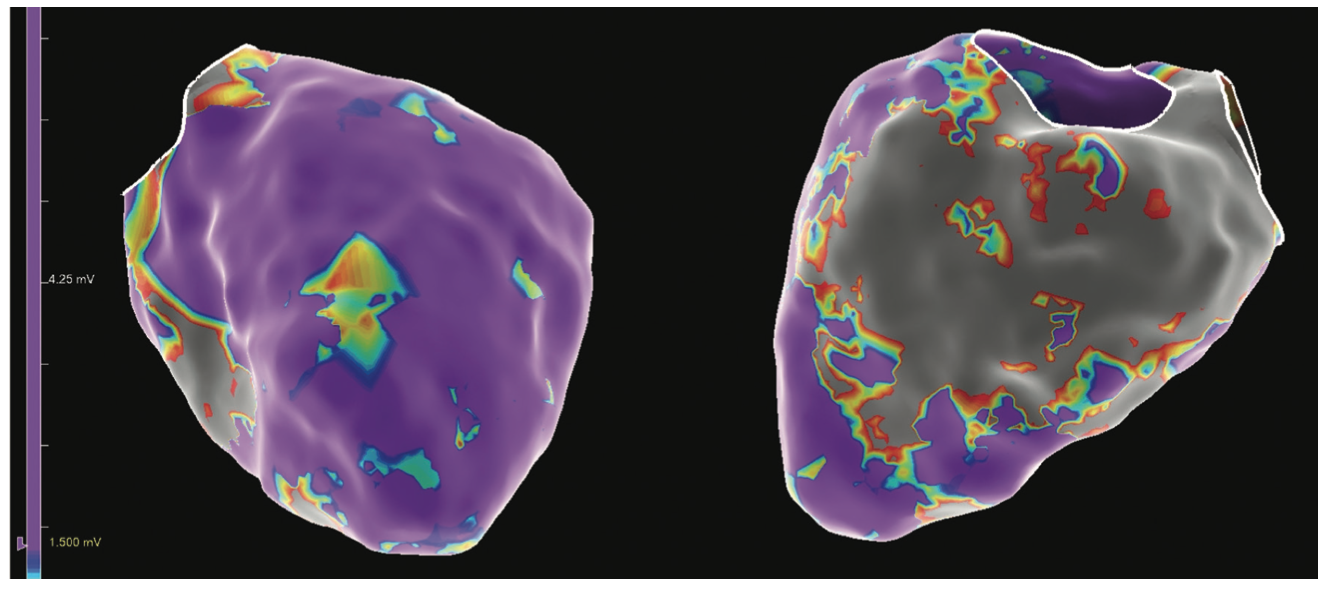
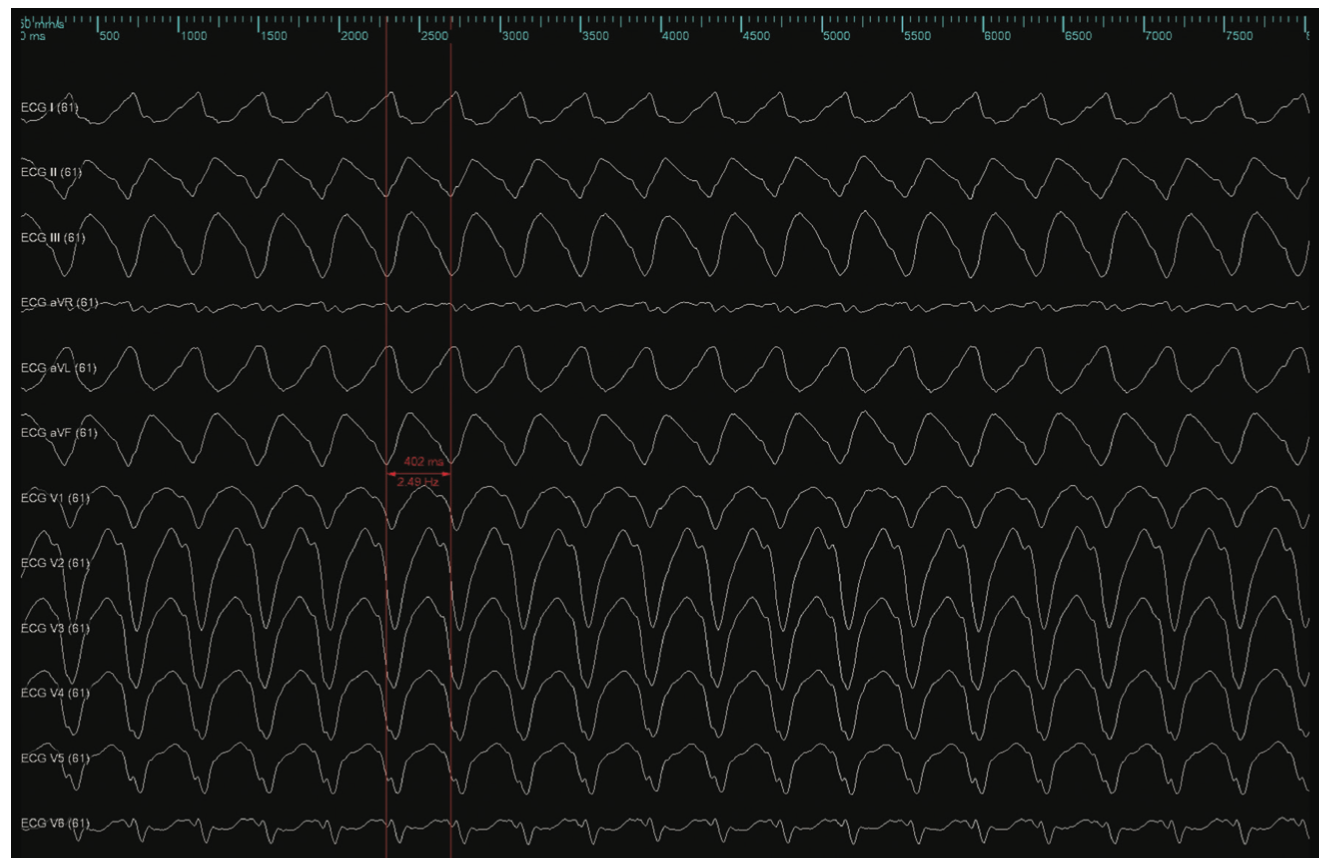
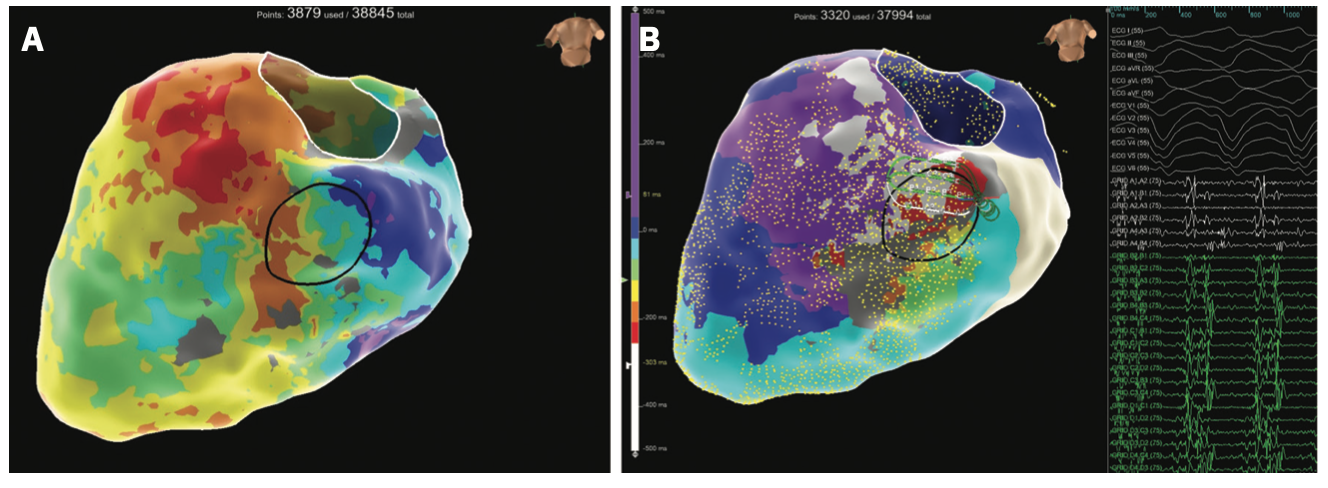
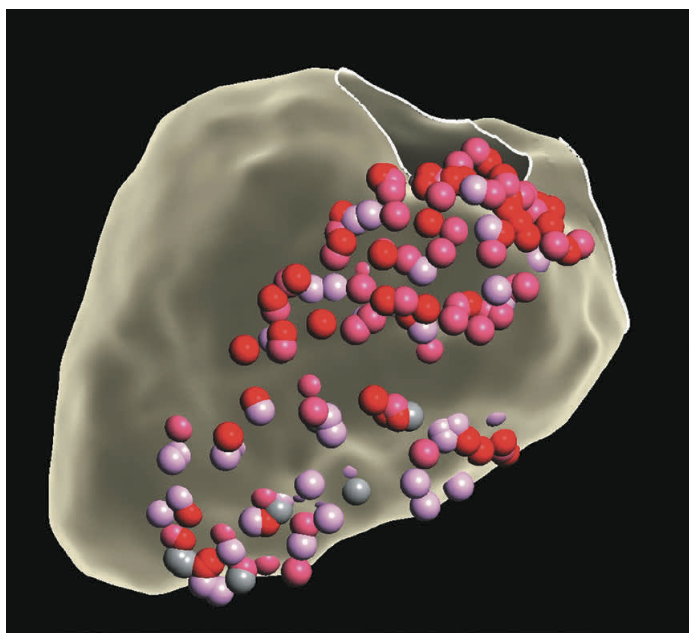
Discussion
Since the pioneering work of Josephson et al on surgical aneurysmectomy to treat VT,1 targeting VAs in areas of anatomic scar has been the hallmark of interventional therapy in structural heart disease. Early catheter-based strategies demonstrated the utility of linear RF ablation lesion sets through infarcted areas toward functional or anatomic barriers to reduce arrhythmic events.2,3 Jais et al4 described a more extensive ablative strategy during SR—a tactic that many centers employ today. Given the morbidity of often long procedures requiring multiple VT inductions, a more extensive substrate-based approach offers obvious advantage to improve the safety and efficacy of catheter-based VT ablation.
In parallel, the development of high-density mapping technology has allowed for more rapid and comprehensive characterization of electroanatomic substrate. Tung et al built upon prior work on macroreentry in structural heart disease5 to broaden understanding of spatial and temporal activation in VT circuits, taking advantage of these powerful mapping tools.6 Complementary data focusing on regions of slow conduction propagating to the latest zone of activation during SR (ie, isochronal late activation mapping [ILAM])7 demonstrated better correlation with successful ablation sites for VT compared with late potential mapping. Traditional views of ischemic VT circuits defined primarily on the endocardial surface have been challenged and highlight the complexities of treating VT in structural heart disease. The case presented here highlights the evolving approach in ischemic and nonischemic patients using complementary information during SR and VT to more precisely target arrhythmia circuits.
There are still many questions with regard to the most effective strategies to treat VAs. Over the past year, additional data have been published that further support a proactive approach to targeting VT with RF ablation, either at the time of ICD implantation or after the first device-treated event.8,9 Each of these trials used a substrate-based approach with VT induction to further enhance efficacy. Where current catheter ablation approaches have reached their limitations, novel ablation modalities such as pulsed field ablation (PFA) and noninvasive stereotactic body radioablation (SBRT) are important areas of investigation.
Radiotherapy for Refractory VT
Catheter-based RF ablation remains the gold standard for the treatment of VT.10 Patients with refractory VT may fail traditional therapy due to inaccessible substrate or high-risk comorbidities precluding repeat ablation. SBRT (or stereotactic ablative radiotherapy) is a method commonly used in the treatment of solid tumors for precise delivery of radiation to tissue, with minimal interaction to adjacent tissue.11 In the first case series, Cuculich et al described use of SBRT (described as cardiac radioablation [CRA]) targeting myocardium by coupling noninvasive electroanatomic mapping with electrocardiographic imaging and multimodality imaging, including computed tomography, transthoracic echocardiography, and cardiac magnetic resonance imaging to precisely localize VT substrate in 5 high-risk patients with refractory VT.12 A dose of 25 Gy ionizing radiation in a single fraction was prescribed over the total targeted volume (Figure 6).12 Following therapy, there was a reduction from 6577 events to 4 events.11 This novel approach led to the prospective phase I/II ENCORE-VT trial demonstrating efficacy with a 97% reduction in ICD shocks and antitachycardia pacing, and improved quality of life. This therapy is thought to be easier on patients; it lasts 5-10 minutes and treatment occurs while the patient is awake, free-breathing, and listening to music. Because it is entirely noninvasive, there have been no procedural adverse events. In some centers, patients are now treated as outpatients after a 10-minute treatment.
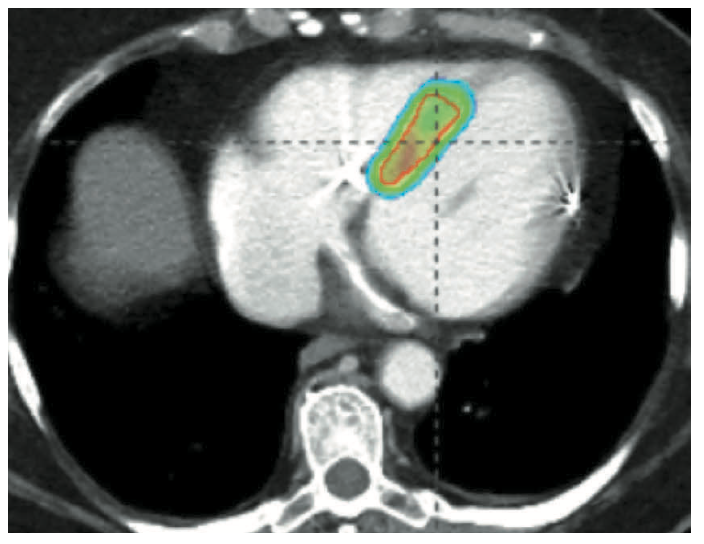
The specific mechanism for CRA therapy is thought to be tissue destruction, similar to thermal ablation from a RF catheter. However, recently published data demonstrated that 25 Gy did not produce transmural scar formation as expected. Moreover, in treated segments, they describe an acute persistent increase in expression of NaV1.5 and Cx43 along with reactivation of Notch signaling (as a radiation response).12 While more study is needed, this upregulation can lead to increased conduction velocity and may represent a mechanism for reentry suppression.13 In a subanalysis of the ENCORE-VT population, spontaneous VT following CRA was not slower, as expected for tissue destruction. Rather, the recurrent VT was either the same rate or faster (93%).14 Several prospective single-arm clinical trials are now underway to establish the efficacy and safety of this therapy. A comparison of CRA vs redo catheter ablation in a randomized control trial is on the near horizon.
The Promise of PFA
An ideal cardiac ablation tool would electrically isolate the targeted substrate by rapidly creating durable full thickness (transmural) lesions while sparing adjacent structures. PFA describes the delivery of multiple short pulses of high voltage between 2 electrodes, creating an electric field that, if strong enough, can create cellular membrane disruption from electroporation, resulting in cell death. Initial preclinical and limited clinical experience demonstrated consistent results during AF ablation, providing improved safety, efficacy, and procedure times.15-22 While less is known regarding the effects of PFA on the ventricular myocardium, the potential for similar procedural results is encouraging.
Koruth et al demonstrated the safety and feasibility of PFA delivery on swine ventricular myocardium using focal catheter ablation to create homogeneous, myocardial-specific lesions with no overlying thrombus while preserving surrounding nerve fascicles and vasculature.22 Im et al compared the lesion characteristics of PFA and RF in healthy and infarcted LV myocardium in swine. In healthy tissue, PFA resulted in greater lesion depth (5.7 + 0.8 mm vs 4.8 + 1.4 mm) and width (11.3 + 1.9 mm vs 8.5 + 1.9 mm), and in contrast to RF ablation, PFA resulted in no evidence of cellular damage to any nerve, artery, or vein. In infarcted tissue, histopathologic measurements demonstrated PFA lesions were significantly deeper than RF ablation (6.1 + 1.7 mm vs 3.8 + 1.7 mm).24 This same group evaluated the effect of epicardial PFA in a swine model and found lesions extended into the myocardium across coronary arteries (CA) and adipose tissue, with a median depth of 4.1 mm. There was a high prevalence of acute CA spasm that spontaneously resolved within 30 minutes, but only minimal to mild chronic stenosis, while sparing epicardial nerves.25
While preclinical ventricular studies show promise, electric field behavior in the ventricle is different than in the atrium, owing to properties including myocardial muscle anisotropy and scar.26 Further research is needed to evaluate tailored parameters involved with PFA during ventricular ablation.27 Mathematical modeling may prove useful to predict lesion geometries with different input parameters, but may not be able to account for the heterogeneous composition of scar or the dynamic changes in tissue conductance during energy delivery.28 To date, there is 1 human case report describing PFA to treat recurrent scar-related VT after prior failed RF ablation. Ouss et al describe overlapping PFA applications in an area of slow conduction within dense scar eliminating near-field electrograms, rendering the patient noninducible and free from arrhythmias at 6 months with no complications.29 Our experience with a variety of PFA technologies treating AF (eg, PulseSelect PFA System, Medtronic; Farapulse PFA, Boston Scientific; and Sphere-9, Affera, a Medtronic company) fuels our excitement about the future of PFA as a cardiac ablation tool, not only in atrial tissue, but possibly for the treatment of VAs as well. Further large-scale clinical trials are needed to fully assess the safety, efficacy, and applicability of PFA to VT ablation.
Disclosure: The authors have completed and returned the ICMJE Form for Disclosure of Potential Conflicts of Interest. They report no conflicts of interest regarding the content herein.
References
1. Martin JL, Untereker W, Harken AH, Horowitz LN, Josephson M. Aneurysmectomy and endocardial resection for ventricular tachycardia: favorable hemodynamic and antiarrhythmic results in patients with global left ventricular dysfunction. Am Heart J. 1982;103(6):960-965. doi:10.1016/0002-8703(82)90557-9
2. Marchlinski F, Callans D, Gottlieb C, Zado E. Linear ablation lesions for control of unmappable ventricular tachycardia in patients with ischemic and nonischemic cardiomyopathy. Circulation. 2000;101(11):1288-1296. doi:10.1161/01.cir.101.11.1288
3. Stevenson WG, Wilber DJ, Natale A, et al. Irrigated radiofrequency catheter ablation guided by electroanatomic mapping for recurrent ventricular tachycardia after myocardial infarction: the multicenter ThermoCool ventricular tachycardia ablation trial. Circulation. 2008;118(25):2773-2782. doi:10.1161/CIRCULATIONAHA.108.788604
4. Jais P, Maury P, Khairy P, et al. Elimination of local abnormal ventricular activities: a new end point for substrate modification in patients with scar-related ventricular tachycardia. Circulation. 2012;125(18):1284-1296. doi:10.1161/CIRCULATIONAHA.111.043216
5. de Bakker JM, van Capelle FJ, Janse MJ, et al. Macroreentry in the infarcted human heart: the mechanism of ventricular arrhythmias with a “focal” activation pattern. J Am Coll Cardiol. 1991;18(4):1005-1014. doi:10.1016/0735-1097(91)90760-7
6. Tung R, Raiman M, Liao H, et al. Simultaneous endocardial and epicardial delineation of 3d reentrant ventricular tachycardia. J Am Coll Cardiol. 2020;75(8):884-897. doi:10.1016/j.jacc.2019.12.044
7. Irie T, Yu R, Bradfield JS, et al. Relationship between sinus rhythm late activation zones and critical sites for scar-related ventricular tachycardia: systematic analysis of isochronal late activation mapping. Circ Arrhythm Electrophysiol. 2015;8(2):390-399. doi:10.1161/CIRCEP.114.002637
8. Tung R, Xue Y, Chen M, et al, for the PAUSE-ICD Investigators. First-line catheter ablation of monomorphic ventricular tachycardia in cardiomyopathy concurrent with defibrillator implantation: the PAUSE-ICD randomized trial. Circulation. 2022;145(25):1839-1849. doi:10.1161/CIRCULATIONAHA.122.060039
9. Della Bella P, Baratto F, Vergara P, et al, for the PARTITA Investigators. Does timing of ventricular tachycardia affect prognosis in patients with an implantable cardioverter-defibrillator? Results from the multicenter randomized PARTITA trial. Circulation. 2022;145(25):1829-1838. doi:10.1161/CIRCULATIONAHA.122.059598
10. Cronin EM, Bogun FM, Maury P, et al. 2019 HRS/EHRA/APHRS/LAHRS expert consensus statement on catheter ablation of ventricular arrhythmias. Heart Rhythm. 2019;17(1):e2-e154. doi:10.1016/j.hrthm.2019.03.002
11. Benedict SH, Yenice KM, Followill D, et al. Stereotactic body radiation therapy: the report of AAPM Task Group 101: stereotactic body radiation therapy: the report of TG101. Med Phys. 2010;37(8):4078-4101. doi:10.1118/1.3438081
12. Cuculich PS, Schill MR, Kashani R, et al. Noninvasive cardiac radiation for ablation of ventricular tachycardia. N Engl J Med. 2017;377(24):2325-2336. doi:10.1056/NEJMoa1613773
13. Zhang DM, Navara R, Yin T, et al. Cardiac radiotherapy induces electrical conduction reprogramming in the absence of transmural fibrosis. Nat Commun. 2021;12(1):5558. doi:10.1038/s41467-021-25730-0
14. Wiener PC, Steyers CM, Moore K, et al. PO-653-07 Ventricular arrhythmias before and after noninvasive cardiac radioablation: a secondary analysis of the ENCORE-VT trial. Heart Rhythm. 2022;19(5 Suppl):S256-S257. doi:10.1016/j.hrthm.2022.03.259
15. du Pré BC, van Driel VJ, van Wessel H, et al. Minimal coronary artery damage by myocardial electroporation ablation. Europace. 2013;15(1):144-149. doi:10.1093/europace/eus171
16. Neven K, van Es R, van Driel V, et al. Acute and long-term effects of full-power electroporation ablation directly on the porcine esophagus. Circ Arrhythm Electrophysiol. 2017;10(5):e004672. doi:10.1161/CIRCEP.116.004672.
17. van Driel VJ, Neven K, van Wessel H, et al. Low vulnerability to the phrenic nerve to electroporation ablation. Heart Rhythm. 2015;12(8):1838-1844. doi:10.1016/j.hrthm.2015.05.012
18. Yavin H, Shapira-Daniels A, Barkagan M, et al. Pulsed field ablation using a lattice electrode for focal energy delivery: biophysical characterization, lesion durability, and safety evaluation. Circ Arrhythmia Electrophysiol. 2020;13(6):e008580. doi:10.1161/CIRCEP.120.008580
19. Reddy VK, Koruth J, Jais P, et al. Ablation of atrial fibrillation with pulsed electric fields: an ultra-rapid, tissue=selective modality for cardiac ablation. JACC Clin Electrophysiol. 2018;4(8):987-995. doi:10.1016/j.jacep.2018.04.005
20. Verma A, Boersma L, Haines D, et al. First-in-human experience and acute procedural outcomes using a novel pulsed field ablation system: the PULSED AF pilot trial. Circ Arrhythm Electrophysiol. 2022;15(1):e010168. doi:10.1161/CIRCEP.121.010168
21. Reddy VY, Neuzil P, Koruth JS, et al. Pulsed field ablation for pulmonary vein isolation in atrial fibrillation. J Am Coll Cardiol. 2019;74(3):315-326. doi:10.1016/j.jacc.2019.04.021
22. Reddy VY, Dukkipati SR, Neuzil P, et al. Pulsed field ablation for atrial fibrillation: 1-year outcomes of IMPULSE, PEFCAT and PEFCAT II. JACC Clin Electrophysiol. 2021;7(5):614-627. doi:10.1016/j.jacep.2021.02.014
23. Koruth JS, Kuroki K, Iwasawa J, et al. Endocardial pulsed field ablation: a proof of concept preclinical evaluation. Europace. 2020;22(3):434-439. doi:10.1093/europace/euz341
24. Im SI, Higuchi S, Lee A, et al. Pulsed field ablation of left ventricular myocardium in a swine infarct model. JACC Clin Electrophysiol. 2022;8(6):722-731. doi:10.1016/j.jacep.2022.03.007
25. Higuchi S, Im SI, Stillson C, et al. Effect of epicardial pulsed field ablation directly on coronary arteries. JACC Clin Electrophysiol. 2022;8(12):1486-1496. doi:10.1016/j.jacep.2022.09.003
26. Xie F, Zemulin CW. Effect of twisted fiber anisotropy in cardiac tissue on ablation with pulsed electric fields. PloS One. 2016;11(4):e0152262. doi:10.1371/journal.pone.0152262
27. Sugrue A, Maor E, Munoz F, et al. Cardiac ablation with pulsed electric fields: principles and biophysics. Europace. 2022;24(8):1213-1222. doi:10.1093/europace/euac033
28. Mehta NK, Simon R, Shah K. PO-718-08 Predicting tissue conductance changes and ablation lesion patterns using quasi-dynamic pulsed field electroporation numerical model for cardiac ablation. Heart Rhythm. 2022;19(5 Suppl):S507. doi:10.1016/j.hrthm.2022.03.1193
29. Ouss A, van Stratum L, van der Voort P, Dekker L. First in human pulsed field ablation to treat scar-related ventricular tachycardia in ischemic heart disease: a case report. J Interv Card Electrophysiol. 2022 Oct 29. doi:10.1007/s10840-022-01407-6