ADVERTISEMENT
Catheter Ablation of Ventricular Tachycardia in the Setting of Ischemic Cardiomyopathy: A Case-Based Approach
Sudden cardiac death is still a significant public health issue with the etiology largely attributed to ventricular arrhythmias such as ventricular tachycardia (VT) and ventricular fibrillation (VF).1 Structural heart disease in the setting of either ischemic or non-ischemic cardiomyopathy can predispose patients to VT. While implantable cardioverter-defibrillators (ICDs) remain the cornerstone of therapy for prevention of sudden death from ventricular arrhythmias, antiarrhythmic drug therapy and catheter ablation have both shown to reduce the incidence of VT/VF.2 Several approaches to VT ablation have been described, and many of these techniques have evolved over the last few years, with advances in imaging, catheter technology, and improved understanding of VT mechanisms.
Our objective in this publication is to present a systematic approach to catheter ablation in a patient presenting with VT in the setting of ischemic heart disease.
Case Presentation
Our patient is a 76-year-old male with a past medical history of coronary artery disease treated with coronary artery bypass grafting and multiple percutaneous coronary interventions, chronic systolic heart failure with a left ventricular ejection fraction (EF) of 30%, and previous cardiac resynchronization therapy-defibrillator. He presented to Loyola University Medical Center with recurrent episodes of symptomatic VT despite antiarrhythmic drug therapy. ICD interrogation showed multiple episodes of VT with a cycle length (CL) of approximately 400 ms, which terminated with antitachycardia pacing or ICD shocks. Transthoracic echocardiogram (TTE) showed a stable EF of 30%, a severely dilated left ventricle, and akinesis of the apex, mid to apical anterior wall, and anteroseptum.
During the electrophysiology study, VT was induced with a single extrastimulus from the right ventricular apex (CL 420 ms, right bundle branch block morphology, mid-inferior axis with precordial concordance; Figure 1). The far-field electrogram (EGM) morphology from the patient’s ICD matched the EGM induced in the EP lab, confirming induction of clinical VT. This was not hemodynamically tolerated, ultimately requiring pace termination. We proceeded with a substrate-based strategy to target the VT using the EnSite Precision cardiac mapping system (Abbott). Voltage mapping during right ventricular pacing demonstrated areas of low voltage in the basal anteroseptum (Figure 2A). Isochronal late activation map (ILAM) demonstrated an area of isochronal crowding/deceleration zone (DZ) on the basal anteroseptum (Figure 2B). Pace mapping (PM) performed in this region was excellent with a calculated match score of 97 (Figure 2C). VT was then induced from this region with pacing. This demonstrated concealed fusion post-pacing interval (PPI) equal to tachycardia cycle length (TCL) (Figure 2D). Limited activation mapping was also performed, demonstrating an inferior direction of wavefront by the mitral annulus. Ablation was performed in the border zone of low voltage on the anterior mitral annulus, specifically in the region of excellent PM and deceleration zones (DZ; Figure 3A). VT was no longer inducible with triple extrastimuli from the right and left ventricles. A post-ablation substrate map was performed demonstrating absence of DZ, late potentials (LP), and local abnormal ventricular activity (LAVA) as additional endpoints (Figure 3B).
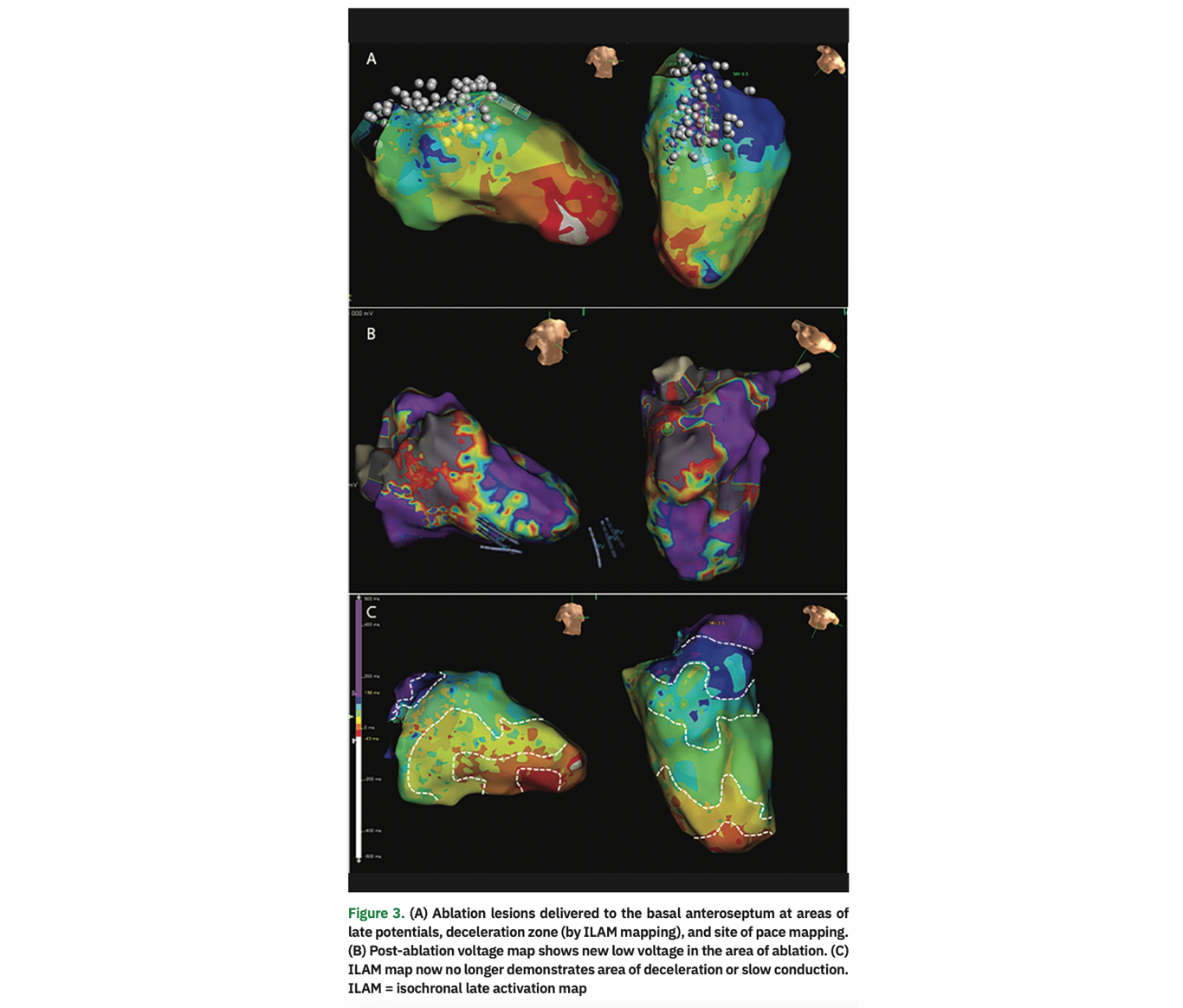
Discussion
Approach to Preprocedural Planning
Preprocedural planning is essential in anticipating procedural findings and challenges, as well as establishing a strategy for ablation. When available, a 12-lead electrocardiogram (ECG) of the VT episode can predict VT exit sites,3 and this can be correlated to structural imaging4,5 in order to determine the areas to focus on during mapping and ablation. Unfortunately, an ECG capturing the VT episode may not be available, particularly in ambulatory patients. In this case, review of far-field EGMs from the patient’s ICD can serve as a template for clinical VT. Subsequently, structural imaging is important to determine location and extent of scar as a potential substrate for arrhythmia. TTE can identify wall motion abnormalities and wall thinning, though dedicated imaging, typically with computed tomography (CT) or magnetic resonance imaging (MRI), can provided more detailed anatomic information for procedural guidance.6-8 Although CT was not performed preprocedurally in this patient, wall thinning observed on CT has been shown to correlate with endocardial low voltage, as well as functional markers of slow conduction such as DZ, LP, and LAVA.9,10 We show a representative example from a different case in Figure 4.
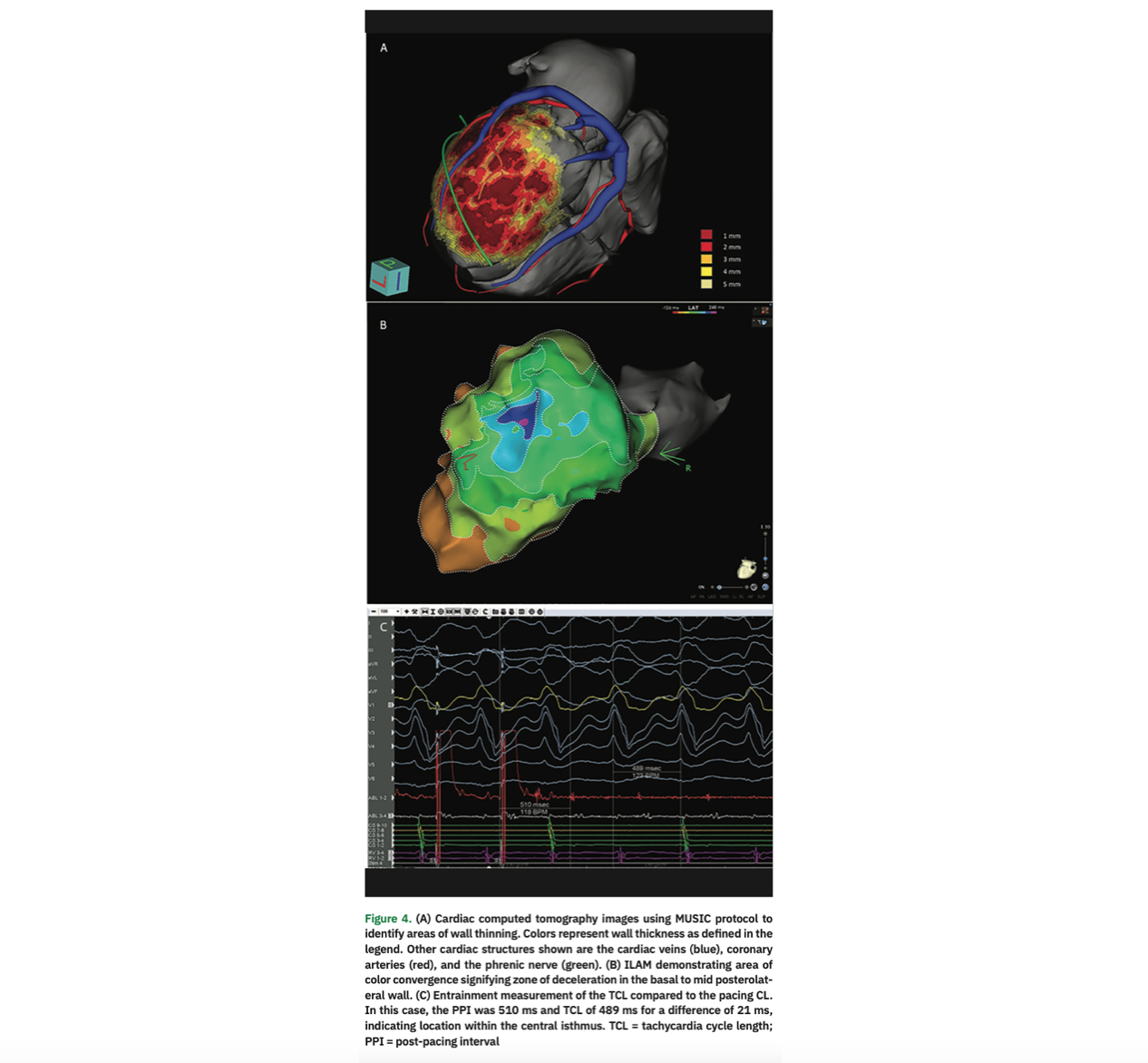
Approaches to Catheter Ablation
The approaches to mapping and ablation use four main techniques: activation mapping, entrainment mapping, substrate mapping, and pace mapping. We discuss these individual approaches below.
Activation Mapping and Entrainment Mapping. Conventional mapping strategies, including creation of the full activation sequence of VT along with entrainment to identify the critical isthmus, remain the gold standard for VT ablation. Unfortunately, these strategies are not feasible, as the majority of induced VT is poorly tolerated; this is attributed to patchy scar in the current early reperfusion era, which typically results in faster VT. In our patient, we were fortunately able to obtain entrainment data as VT was induced with pacing at the site of interest (as determined by an area of excellent PM, scar border zone, and DZ). This demonstrated concealed fusion with PPI equal to TCL. The stimulus to QRS (S-QRS) interval was short (<30% TCL), indicating entrainment close to the exit site. Although an activation map is not essential for a substrate-based strategy, in this case, limited activation mapping identified the directionality of VT wavefront. We were then able to extrapolate the approximate site of the critical isthmus proximal to the exit site, combining the information gathered from the DZ, PM, EGM, S-QRS, and voltage/border zone, as discussed below.
Substrate Mapping. If the induced VT is not hemodynamically tolerated, we will proceed with a substrate-based strategy, as we did primarily in this case. Used in conjunction with imaging obtained as part of preprocedural planning, mapping of the substrate with voltage can help identify sites of scar that may participate in re-entrant VT. In the left ventricle, standard values of >1.5 mV are used to delineate healthy myocardium and values less than 0.5 mV are considered to be dense scar.11 Critical isthmuses of VT circuits are almost always located in areas of low voltage. One advantage of substrate mapping is that it can be done in sinus or paced rhythm in cases when VT is not stable or non-inducible. Various strategies of substrate mapping and ablation have been described. While in-depth description of each technique is beyond the scope of this report, these techniques are widely utilized and include ablation of LP, scar homogenization/LAVA elimination, scar dechanneling, and core isolation.8,11-16 Additionally, ILAM mapping, which was recently described, aims to identify areas of slow or delayed activation or DZ, using color-coded areas that each occupy an identical portion of the CL activation time, termed isochrones, during sinus rhythm or ventricular pacing. Areas that include >3 isochrones in a 1-cm radius indicate deceleration zones that may harbor the critical components of the VT circuit.17 In our case, a single area of DZ was noted at the anterior mitral annulus. This was in proximity to the exit site based on PM (discussed below) at the scar border in the region of LP/LAVA. Targeted ablation of the DZ has been described as an endpoint of VT ablation. We will aim to ablate all identified DZ (only a single DZ in this case) as an endpoint for ablation, in addition to elimination of LP/LAVA along with VT non-inducibility.
Pace Mapping. In our approach to VT ablation, we will always initially attempt to induce VT. The device EGM of the induced VT is compared to the stored EGM of the clinical VT to ensure induction of clinical VT (especially if a 12-lead ECG of the clinical VT is not available). For hemodynamically tolerated VT, we will resort to conventional mapping strategies such as activation/entrainment mapping. If VT is unstable or not inducible, we will rely on PM as one of the surrogates to identify the exit site of VT. Pacing is performed in sinus rhythm and the paced QRS is quantitively compared to the QRS template of the induced VT.18 Morphologic differences between paced complexes and the clinical QRS can also help guide the relative position of the catheter to the exit site.19,20 In our case, the pace match score was excellent, suggesting pacing from the exit site of the VT. Additional data from DZ, voltage map, and LP/LAVA electrograms (along with activation map or entrainment data if available) is then all compiled to determine the site of ablation, as demonstrated in this case.
Conclusion
We present an approach to invasive management of a patient with ischemic cardiomyopathy and recurrent VT and ICD therapies despite antiarrhythmic pharmacotherapy. Preprocedural planning is essential to help guide the localization of VT and identify potential areas of substrate from which it may originate. ECG and ICD interrogation data can identify clinical VTs, and additional imaging can identify areas of wall thinning and scarring that will be the focus of mapping and ablation. We chose to describe a case where VT was hemodynamically not tolerated, and how surrogate endpoints such as voltage, PM, DZ, LP, and LAVA can help identify the critical components of the VT circuit. Our general approach to VT ablation is presented in an algorithmic format in Figure 5.
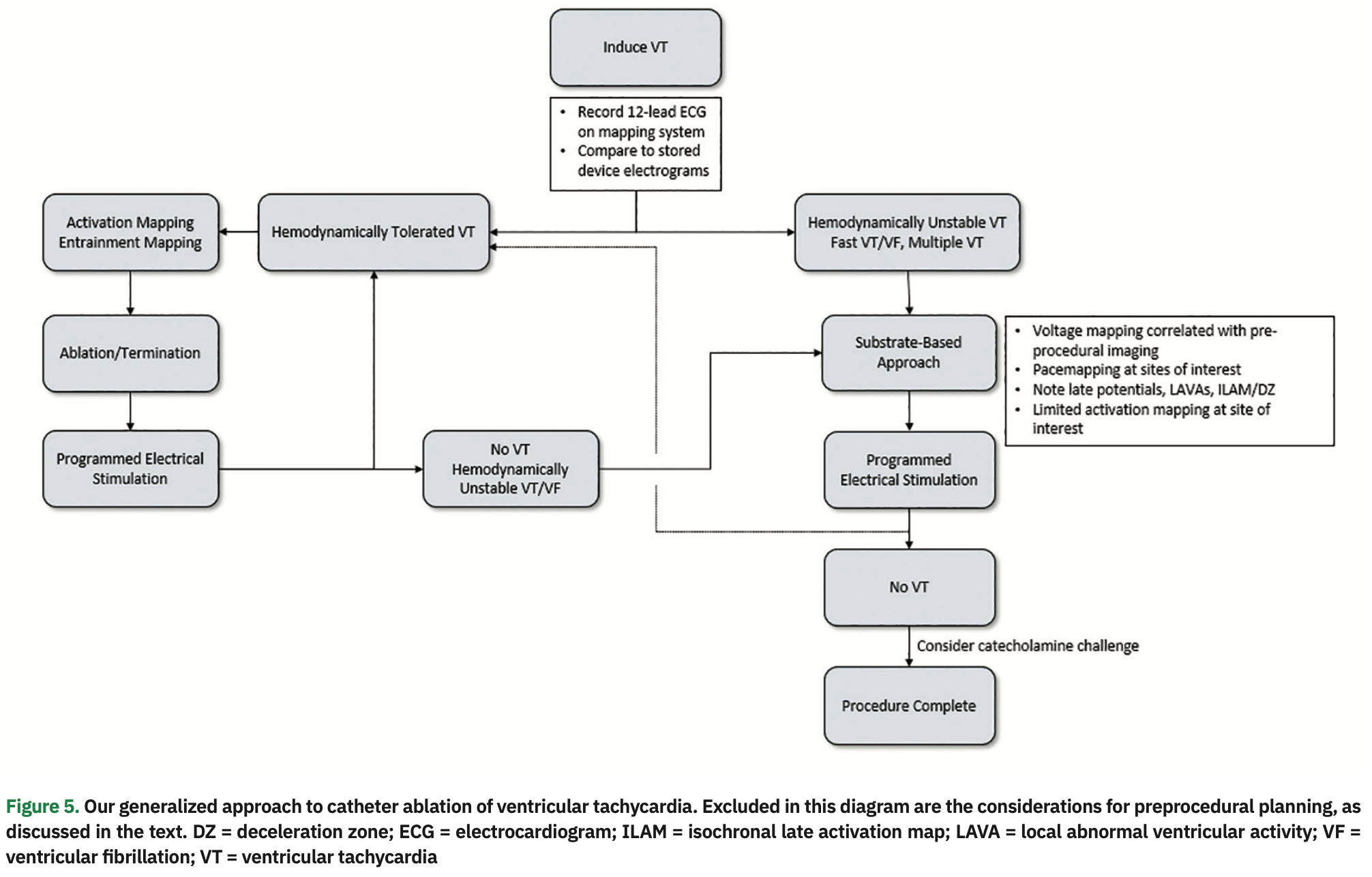
Disclosures: The authors have completed and returned the ICMJE Form for Disclosure of Potential Conflicts of Interest. Dr. Santucci reports consulting fees from Biosense Webster and participation on a Data Safety Monitoring Board or Advisory Board for St. Jude/Abbott. The remaining authors have no conflicts of interest to report regarding the content herein.
References
1. Guandalini GS, Liang JJ, Marchlinski FE. Ventricular tachycardia ablation: past, present, and future perspectives. JACC Clin Electrophysiol. 2019;5(12):1363-1383. doi: 10.1016/j.jacep.2019.09.015
2. Sears SF, Conti JB. Quality of life and psychological functioning of ICD patients. Heart. 2002;87(5):488-493. doi: 10.1136/heart.87.5.488
3. Josephson ME, Horowitz LN, Waxman HL, et al. Sustained ventricular tachycardia: role of the 12-lead electrocardiogram in localizing site of origin. Circulation. 1981;64(2):257-272. doi: 10.1161/01.cir.64.2.257
4. Piers SRD, Tao Q, Van Huls Van Taxis CFB, Schalij MJ, Van Der Geest RJ, Zeppenfeld K. Contrast-enhanced MRI-derived scar patterns and associated ventricular tachycardias in nonischemic cardiomyopathy: implications for the ablation strategy. Circ Arrhythmia Electrophysiol. 2013;6(5):875-883. doi: 10.1161/CIRCEP.113.000537
5. White JA, Fine NM, Gula L, et al. Utility of cardiovascular magnetic resonance in identifying substrate for malignant ventricular arrhythmias. Circ Cardiovasc Imaging. 2012;5(1):12-20. doi: 10.1161/CIRCIMAGING.111.966085
6. Piers SR, Zeppenfeld K. Imaging-guided ventricular tachycardia ablation. Arrhythmia Electrophysiol Rev. 2013;2(2):128-134. doi: 10.15420/aer.2013.2.2.128
7. Mahida S, Sacher F, Dubois R, et al. Cardiac imaging in patients with ventricular tachycardia. Circulation. 2017;136(25):2491-2507. doi: 10.1161/CIRCULATIONAHA.117.029349
8. Yamashita S, Sacher F, Mahida S, et al. Image integration to guide catheter ablation in scar-related ventricular tachycardia. J Cardiovasc Electrophysiol. 2016;27(6):699-708. doi: 10.1111/jce.12963
9. Tian J, Jeudy J, Smith MF, et al. Three-dimensional contrast-enhanced multidetector CT for anatomic, dynamic, and perfusion characterization of abnormal myocardium to guide ventricular tachycardia ablations. Circ Arrhythmia Electrophysiol. 2010;3(5):496-504. doi: 10.1161/CIRCEP.109.889311
10. Komatsu Y, Cochet H, Jadidi A, et al. Regional myocardial wall thinning at multidetector computed tomography correlates to arrhythmogenic substrate in postinfarction ventricular tachycardia: assessment of structural and electrical substrate. Circ Arrhythmia Electrophysiol. 2013;6(2):342-350. doi: 10.1161/CIRCEP.112.000191
11. Marchlinski FE, Callans DJ, Gottlieb CD, Zado E. Linear ablation lesions for control of unmappable ventricular tachycardia in patients with ischemic and nonischemic cardiomyopathy. Circulation. 2000;101(11):1288-1296. doi: 10.1161/01.cir.101.11.1288
12. Arenal A, Glez-Torrecilla E, Ortiz M, et al. Ablation of electrograms with an isolated, delayed component as treatment of unmappable monomorphic ventricular tachycardias in patients with structural heart disease. J Am Coll Cardiol. 2003;41(1):81-92. doi: 10.1016/s0735-1097(02)02623-2
13. Jaïs P, Maury P, Khairy P, et al. Elimination of local abnormal ventricular activities: a new end point for substrate modification in patients with scar-related ventricular tachycardia. Circulation. 2012;125(18):2184-2196. doi: 10.1161/CIRCULATIONAHA.111.043216
14. Di Biase L, Santangeli P, Burkhardt DJ, et al. Endo-epicardial homogenization of the scar versus limited substrate ablation for the treatment of electrical storms in patients with ischemic cardiomyopathy. J Am Coll Cardiol. 2012;60(2):132-141. doi: 10.1016/j.jacc.2012.03.044
15. Berruezo A, Fernández-Armenta J, Mont L, et al. Combined endocardial and epicardial catheter ablation in arrhythmogenic right ventricular dysplasia incorporating scar dechanneling technique. Circ Arrhythmia Electrophysiol. 2012;5(1):111-121. doi: 10.1161/CIRCEP.110.960740
16. Tzou WS, Frankel DS, Hegeman T, et al. Core isolation of critical arrhythmia elements for treatment of multiple scar-based ventricular tachycardias. Circ Arrhythmia Electrophysiol. 2015;8(2):353-361. doi: 10.1161/CIRCEP.114.002310
17. Aziz Z, Shatz D, Raiman M, et al. Targeted ablation of ventricular tachycardia guided by wavefront discontinuities during sinus rhythm: a new functional substrate mapping strategy. Circulation. 2019;140(17):1383-1397. doi: 10.1161/CIRCULATIONAHA.119.042423
18. Gerstenfeld EP, Dixit S, Callans DJ, Rajawat Y, Rho R, Marchlinski FE. Quantitative comparison of spontaneous and paced 12-lead electrocardiogram during right ventricular outflow tract ventricular tachycardia. J Am Coll Cardiol. 2003;41(11):2046-2053. doi: 10.1016/s0735-1097(03)00427-3
19. De Chillou C, Groben L, Magnin-Poull I, et al. Localizing the critical isthmus of postinfarct ventricular tachycardia: the value of pace-mapping during sinus rhythm. Heart Rhythm. 2014;11(2):175-181. doi: 10.1016/j.hrthm.2013.10.042
20. Tung R, Mathuria N, Michowitz Y, et al. Functional pace-mapping responses for identification of targets for catheter ablation of scar-mediated ventricular tachycardia. Circ Arrhythmia Electrophysiol. 2012;5(2):264-272. doi: 10.1161/CIRCEP.111.967976