ADVERTISEMENT
Understanding Surgical Innovations Within Foot and Ankle Surgery
During childhood, and even as an adult, most of us at some point dreamt of or desired the superpower ability of X-ray vision—specifically, the ability to see through inanimate objects, whether for curiosity, pleasure, efficiency, or to improve decision making capabilities with life choices. This desire has not diminished when it comes to surgical innovations within the foot and ankle surgical subspecialty. No matter if we are talking about the radiolucent operating room table, surgical instrumentation, or definitive fixation, we need the ability to see through certain objects in order to ascertain a better view of the most important ones.
Enter radiolucent plating technology. Ultimately, foot and ankle surgeons need the ability to directly visualize the osseous or joint structures they wish to reconstruct. This visualization can influence intraoperative and postoperative decision making. For example, when anatomically reducing a fracture, postoperative outcomes have been directly correlated with how close a surgeon can restore anatomic alignment. If the surgeon utilizes a traditional metallic plate, this can potentially block visualization of the fracture on certain radiographic views.
In comparison, using radiolucent plating fixation provides the surgeon with uninhibited views of the fracture within all cardinal planes for better intraoperative evaluation of fracture reduction. An additional common elective pedal surgical procedure example is when utilizing radiolucent plating fixation during first metatarsophalangeal joint (MTPJ) arthrodesis. The literature demonstrates that an oblique lag screw with a dorsal plate is the strongest biomechanical fixation construct.1-2
If the plate is also radiolucent, it then offers the additional benefit of improved visualization of the fusion site. If surgeons can directly see their unobstructed intraoperative alignment and compression of the great toe joint, then this permits improved surgeon self-critical assessment at the key point-of-care moment during surgery when there’s still an opportunity for change. Likewise, if surgeons can directly visualize osseous bridging on the postoperative AP foot radiograph (instead of having it obscured by a dorsal metallic plate), then they can make more informed decisions on a patient’s weight-bearing status, return to shoe gear, activity, employment, or even athletics (Figure 1).
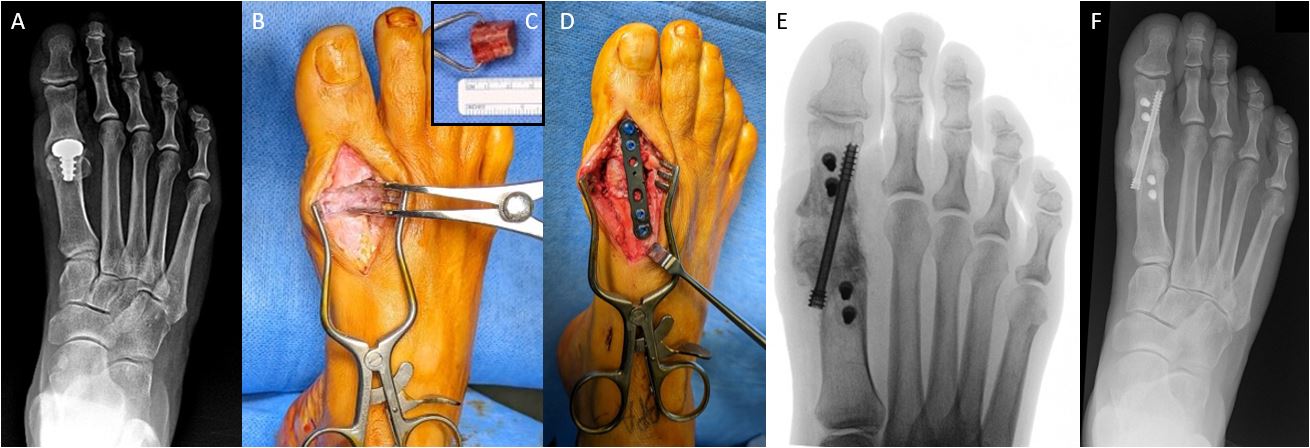
Traditionally, metallic plating has certainly been a workhorse for many traumatic injuries, along with elective reconstructive foot and ankle surgical procedures. However, innovation has the potential to bring additional opportunities for improved materials, create new surgeon efficiencies, and ultimately produce potentially superior patient outcomes.
What You Should Know About CFR-PEEK
What if there were a fixation material that could provide the same reliable stability and reproducibility as metallic plating, but carried additional benefits, even beyond radiolucency? There is. Enter carbon fiber-reinforced polyetheretherketone (CFR-PEEK). The majority of this author’s (BPA) experience with CFR-PEEK has been with the CoLink® PCR plating systems (ConMed Foot and Ankle), which have been successfully utilized in elective as well as traumatic foot and ankle surgical procedures since its market introduction a couple of years ago.
CFR-PEEK’s radiolucency may be the most attractive property for surgeons; however, it carries many significant additional benefits. CFR-PEEK has superior fatigue performance when compared to identical devices manufactured from titanium alloys. This is due to the alternating directions of layered carbon fiber.3
CFR-PEEK’s modulus of elasticity can be altered to be closer to native bone and thus leads to less stress shielding than stiffer traditional metallic plating counterparts.4 This results in the osseous structure healing better and stronger for the patient. There is no cold-welding effect like one may encounter with traditional metallic plating. Consequently, if the hardware ever needs to be removed, this can make for a safer and easier removal process.
CFR-PEEK is extremely biocompatible with human soft tissues and bone, resulting in significantly less inflammatory reaction compared to metal, including the absence of corrosion or wear debris.5-7 Additionally, the material is inert and the literature does not currently have any patient reports of allergic reactions to this implanted material. Finally, CFR-PEEK is compatible with advanced imaging, including computed tomography (CT) and magnetic resonance imaging (MRI). This eliminates or reduces the potential for scatter artifact, a common barrier encountered with traditional metallic plating, if patients ever require advanced imaging of their lower extremity in the future.
A Closer Look at the Literature and Surgeon’s Experience
Two recent systematic reviews published in 2022 and 2023 sought to further analyze modern day use of CFR-PEEK. The first evaluated its use in extremity trauma (proximal humerus, distal radius, distal femur, and ankle fractures). The latter evaluated complications associated with carbon fiber plate fixation compared to metal plates. Results from both studies demonstrated high union rates, low complication rates, with noted advantages over metal (as mentioned earlier in this article), and concluded that CFR-PEEK is a valid alternative over conventional metallic plating.8-9
One unique benefit that this author (BPA) has personally experienced is the radiolucent material’s ability to establish, build, and grow the surgeon-patient trust relationship. In that regard, radiolucent material goes well beyond being a gimmick. This trust factor can be difficult to quantify or measure beyond patient satisfaction scores and subjective feedback, but a surgeon’s ability to demonstrate a patient’s progressive osseous healing elicits confidence from the patient towards their surgeon.
Postoperatively, this has potential to also influence decisions within the recovery timeline related to safe return to weightbearing activity, driving, employment, and sports activity (Figure 2). In contrast, a surgeon illustrating an obstructing metal plate to the patient on their radiographs may not elicit the same positive response. Instead, a “metal-phobia” type response may elicit anxiety about retained metal in the body or the potential need for a secondary procedure and hardware removal.
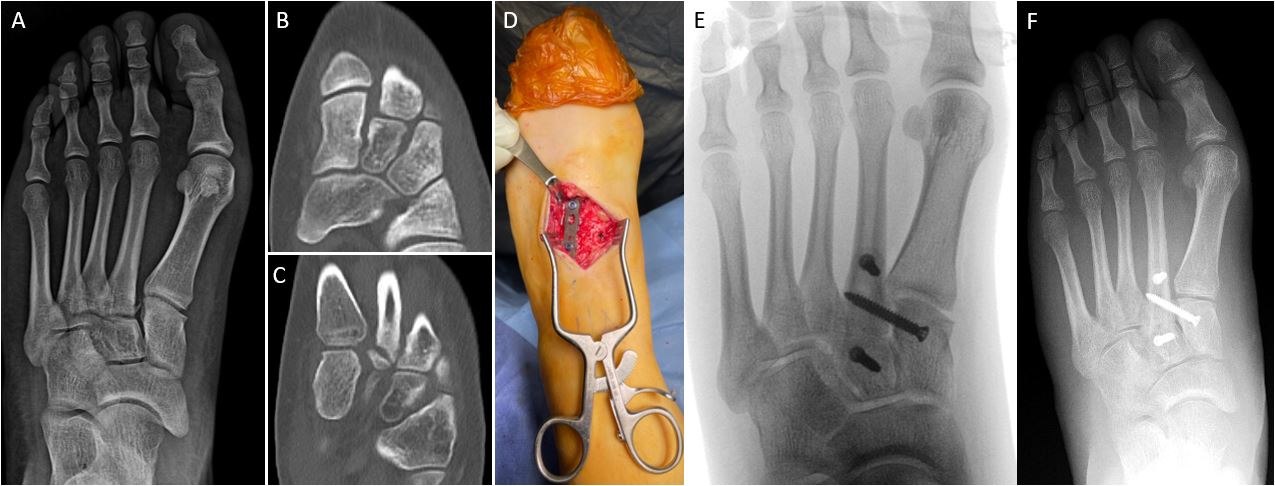
Despite the attractiveness of its radiolucent properties and the additional aforementioned benefits, CFR-PEEK plating utilization remains in the minority compared to metallic alternatives. Even scarcer is the body of literature reporting on CFR-PEEK’s use within elective foot and ankle surgery. The majority of the current literature focuses on its use in orthopedic oncology due to its usefulness when monitoring for recurrent bone tumors. Additionally, there is a growing body of CFR-PEEK use in extremity trauma, including specifically for ankle fractures.
Based on this evidence and our experience, we believe the future will see expanded use of this material within elective foot and ankle surgery, and there exists an enormous opportunity for product development, innovation, and ultimately improving patient care outcomes. Further research should focus on comparative studies against metallic counterparts, but currently available outcomes utilizing CFR-PEEK continue to demonstrate very promising results.
X-ray vision is no longer a superpower that foot and ankle surgeons can only dream about, but options currently exist allowing us to employ this type of fixation in our elective and traumatic reconstructive osseous procedures with many benefits for surgeons and patients that extend well beyond its radiolucent property.
Can PSI and 3D Printing Innovate Foot and Ankle Surgery?
With the continuous evolution of technology, patient-specific instrumentation (PSI) and additive manufacturing, commonly referred to as 3D printing, are emerging as transformative tools in the surgical management of foot and ankle conditions. These advancements are redefining surgical practices, providing new opportunities for personalized treatment approaches, and enhancing patient outcomes by addressing specific anatomical challenges more accurately than ever before.
PSI represents a departure from traditional, one-size-fits-all surgical instrumentation towards tools that are specifically designed to fit the unique anatomy of individual patients. PSI utilizes imaging data from modalities such as computed tomography (CT) or magnetic resonance imaging (MRI) to create customized surgical guides and instruments.10 These patient-specific tools are designed to align precisely with the patient’s unique anatomical landmarks, facilitating more accurate placement of implants, screws, or other devices.
A major advantage of PSI is the ability to improve the accuracy of implant positioning.10-12 In complex foot and ankle surgeries—such as total ankle arthroplasty, deformity corrections, or complex reconstruction—accurate positioning of implants is critical to achieving optimal clinical outcomes. Misalignment or improper positioning of implants can result in suboptimal function, increased wear, or necessitate revision surgery. PSI leverages preoperative planning based on high-resolution 3-dimensional imaging to create customized surgical guides that are specifically tailored to the patient’s anatomy. These guides assist surgeons in achieving optimal implant positioning and alignment, thereby reducing the risk of complications and improving long-term outcomes (Figure 3).
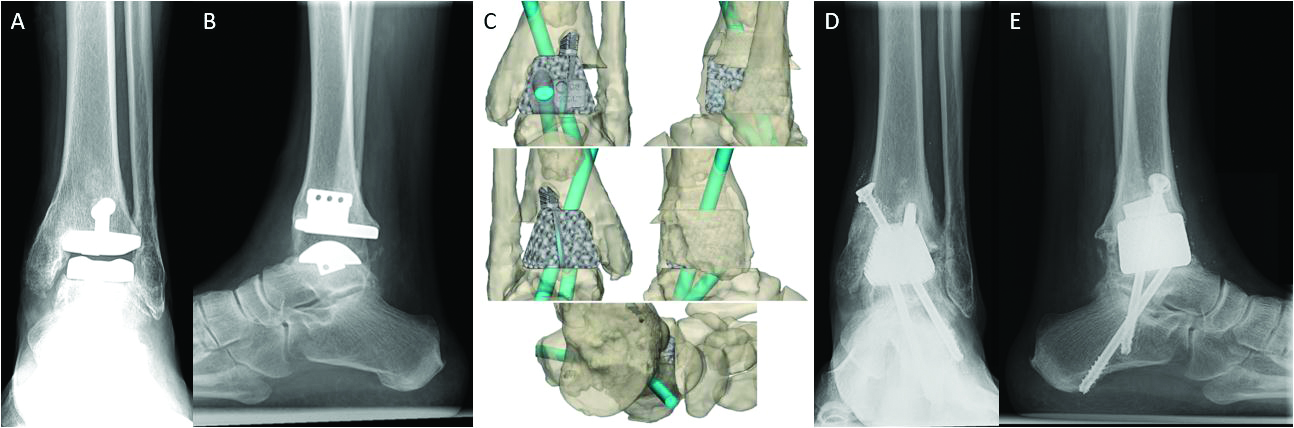
Integrating additive manufacturing into foot and ankle surgery has further augmented the advantages of PSI. Additive manufacturing allows for the creation of highly customized implants and surgical guides that conform precisely to the patient’s anatomical structures—a level of customization that is not feasible with traditional manufacturing techniques. The process begins with the conversion of patient-specific imaging data into a digital model, which is subsequently utilized to design the implant or instrument guides. This digital model then prints layer by layer, resulting in a precise and patient-specific implant.10 This approach allows for the creation of implants that are uniquely suited to each patient’s anatomy, which can be particularly beneficial in cases where patients have unique anatomical challenges, critical-sized bone defects or deformities.
In addition to improving the accuracy of implant placement, 3D printing and PSI offer several other advantages, one of which is the potential for reduced surgical time.13 With PSI, much of the planning and decision-making happens preoperatively, allowing the surgeon to perform the procedure more efficiently.14 This can lead to shorter anesthesia times, reduced risk of infection, and lower overall healthcare costs. The use of patient-specific guides can also reduce the need for intraoperative imaging, which minimizes radiation exposure for both the patient and the surgical team.15
Another advantage is the potential for better clinical outcomes. Studies have shown that the use of PSI and 3D-printed implants can lead to improved alignment, reduced revision rates, and faster recovery times. For example, in total ankle arthroplasty patient-specific guides have been associated with more accurate positioning of the implant components, which can enhance joint function and implant longevity.11-14 Similarly, in complex deformity correction cases, the application of 3D-printed implants can provide a more tailored surgical solution, potentially resulting in improved functional outcomes and higher levels of patient satisfaction.
The ability to create customized implants also broadens the scope of treatment options available to patients. Traditional implants are typically manufactured in a limited range of sizes and shapes, posing challenges in cases where a patient’s anatomical features do not conform to these standardized options. Additive manufacturing enables the production of implants that precisely match the patient’s anatomy, even in scenarios involving severe bone loss, deformities, or other complex anatomical considerations. The literature has recently seen increased case reports and small case series highlighting 3D-printed implants being utilized to address significant osseous defects due to infection, avascular necrosis, trauma, and malignancies.15–17
PSI and additive manufacturing are particularly valuable in revision surgeries, where critical-sized defects arise.18 In revision cases, the patient’s anatomy is significantly altered by prior surgical interventions, making it difficult to achieve an optimal fit with standard implants or structural allografts. Customized implants can be designed to accommodate these unique anatomical changes, providing a better fit and potentially improving the success rates of revisional procedures. Physicians are now able to use 3D-printed porous implants to address patients in whom they had not previously considered salvage options due to critical-sized bone defects.18-20
Challenges With and Questions About 3D Printing and PSI
Despite their numerous benefits, there are challenges and limitations associated with the adoption of PSI and additive manufacturing in foot and ankle surgery. One of the primary challenges is cost. The development and production of patient-specific instruments and implants require significant investment in advanced imaging, software, and additive manufacturing equipment. Additionally, the process of creating these customized devices is time-intensive and requires close collaboration between multidisciplinary teams, including surgeons, radiologists, biomedical engineers, and manufacturing specialists.21-22 These factors can contribute to increased costs, which insurance providers may not fully reimburse.
Regulatory considerations also pose challenges to the widespread adoption of PSI and additive manufacturing. The development and utilization of customized implants must comply with stringent regulatory standards to ensure patient safety and device efficacy. Regulatory bodies require extensive testing and validation of new devices, which can be a lengthy and costly process.23 Additionally, the use of patient-specific implants raises questions regarding liability, intellectual property, and reimbursement that must be carefully navigated.
While early clinical outcomes with PSI and 3D-printed implants are promising, further research is needed to fully understand the long-term impact of these technologies on patient outcomes. Most studies have focused on short- to mid-term results—which, although encouraging, do not provide a complete picture of the potential benefits and risks over an extended period. Continued research, including randomized controlled trials and long-term follow-up studies, is necessary to establish the durability, safety, and effectiveness of patient-specific implants across different patient populations.
The adoption of these new technologies also requires a learning curve. Surgeons must become proficient in digital planning tools, imaging software, and the principles of additive manufacturing to effectively integrate PSI and 3D printing into clinical practice. This necessitates investment in education and training, as well as a willingness to adapt to new surgical techniques and workflows.
Despite these challenges, the future of PSI and additive manufacturing in foot and ankle surgery is promising. Technological advancements are likely to reduce the costs associated with 3D printing, making these customized solutions more accessible to a broader patient population. As more long-term data become available, the use of PSI and additive manufacturing is expected to gain wider acceptance as a standard of care in foot and ankle surgery.
Final Thoughts
Patient-specific instrumentation and additive manufacturing are revolutionizing the field of foot and ankle surgery. These technologies provide an unprecedented level of customization and precision, enabling surgeons to address complex anatomical challenges with greater accuracy and predictability. Although there are challenges related to cost, regulatory approval, and the need for more extensive data, the potential benefits for patients are substantial. As these technologies continue to evolve and integrate into routine clinical practice, they hold the potential to significantly improve surgical outcomes and patient quality of life.
Bradley P. Abicht, DPM, FACFAS, is a board-certified foot and ankle surgeon and Podiatry Department Chair at emplify Health by Gundersen in La Crosse, WI. He completed residency training through the Gundersen Medical Foundation Podiatry Residency. He is an active attending for the current residency program, involved in research, and has clinical interests in percutaneous and minimally invasive surgical techniques, foot and ankle arthroscopy, as well as foot and ankle reconstructive surgery.
Scott Carrington, DPM, FACFAS, is a fellowship-trained board-certified foot and ankle surgeon at emplify Health by Gundersen in La Crosse, WI. He completed his residency training at Presbyterian St. Lukes in Denver, CO followed by advanced specialty training in foot and ankle reconstruction through fellowship at Coordinated Health Orthopedics in Bethlehem, PA. He is an active attending physician with the Gundersen Medical Foundation Podiatry Residency, involved in research, and has clinical interests in post-traumatic reconstruction of the foot and ankle, ankle replacement surgery, arthroscopy, and cartilage restoration.
Bradley P. Abicht, DPM, FACFAS is a consultant for ConMed Foot and Ankle and Invibio.Scott Carrington, DPM, FACFAS is a consultant for ConMed Foot and Ankle.
References
1. Molloy S, Burkhart BG, Jasper LE, Solan MC, Campbell JT, Belkoff SM. Biomechanical comparison of two fixation methods for first metatarsophalangeal joint arthrodesis. Foot Ankle Int. 2003;24(2):169-171.
2. Politi J, John H, Njus G Bennett GL, Kay DB. First metatarsal-phalangeal joint arthrodesis: a biomechanical assessment of stability. Foot Ankle Int. 2003;24(4):332-337.
3. Data on file at In2Bones. Testing data is specific to the CoLink PCR MTP Plate. Mechanical Benchmark of Carbon Fiber PEEK-OPTIMA Ultra-Reinforced vs Ti 6AI-4V Plates undergoing Static and Dynamic Testing per ASTM F382-99 (2008).
4. Hillock R, Howard S. Utility of carbon fiber implants in orthopedic surgery: literature review. Reconstructive Review. March 2014;4(1):23-32.
5. Pinter ZW, Smith KS, Hudson PW, et al. A retrospective case series of carbon fiber plate fixation of ankle fractures. Foot Ankle Spec. 2018;11(3):223-229. doi:10.1177/1938640017718343
6. Behrendt P, Kruse E, Klüter T, et al. Fixed angle carbon fiber reinforced polymer composite plate for treatment of distal radius fractures: pilot study on clinical applications. Unfallchirurg. 2017;120(2):139-146.
7. Rotini R, Cavaciocchi M, Fabbri D, et al. Proximal humeral fracture fixation: multicenter study with carbon fiber peek plate. Musculoskelet Surg. 2015;99(suppl 1):S1-S8.
8. Chloros GD, Prodromidis AD, Wilson J, Giannoudis PV. Fracture fixation in extremity trauma with carbon fiber-reinforced polyetheretherketone (CFR-PEEK) plates: evidence today. Eur J Trauma Emerg Surg. 2022;48(3):2387-2406. doi:10.1007/s00068-021-01778-x
9. Rijs Z, Weekhout A, Daniel S, et al. Carbon-fibre plates for traumatic and (impending) pathological fracture fixation: Where do we stand? A systematic review. J Orthop Traumatol. 2023;24(1):42. Published 2023 Aug 11. doi:10.1186/s10195-023-00724-4
10. Wixted CM, Peterson JR, Kadakia RJ, Adams SB. Three-dimensional printing in orthopaedic surgery: current applications and future developments. J Am Acad Orthop Surg Glob Res Rev. 2021 Apr 20;5(4):e20.00230-11
11. Mazzotti A, Arceri A, Zielli S, Bonelli S, Viglione V, Faldini C. Patient-specific instrumentation in total ankle arthroplasty. World J Orthop. 2022 Mar 18;13(3):230-237. doi: 10.5312/wjo.v13.i3.230
12. Hsu AR, Davis WH, Cohen BE, Jones CP, Ellington JK, Anderson RB. Radiographic outcomes of preoperative CT scan-derived patient-specific total ankle arthroplasty. Foot Ankle Int. 2015;36:1163–1169.
13. Yau J, Emmerson B, Kakwani R, Murty AN, Townshend DN. Patient-reported outcomes in total ankle arthroplasty: patient specific versus standard instrumentation. Foot Ankle Spec. 2024 Feb;17(1_suppl):30S-37S.
14. Arceri A, Abdi P, Mazzotti A, et al. Standard total ankle arthroplasty vs. patient-specific instrumentation: a comparative study. J Personalized Med. 2024; 14(7):770.
15. Cui Y, Chen B, Wang G, et al. Partial talar replacement with a novel 3D printed prosthesis. Comput Assist Surg (Abingdon). 2023;28(1):2198106. doi:10.1080/24699322.2023.2198106
16. Li Z, Lu M, Zhang Y, et al. Reconstruction of the proximal radius with 3D-printed personalized prosthesis after tumor resection: case series. J Shoulder Elbow Surg. 2024;33(3):556-563. doi:10.1016/j.jse.2023.08.032
17. Chen Z, Xing Y, Li X, et al. 3D-printed titanium porous prosthesis combined with the Masquelet technique for the management of large femoral bone defect caused by osteomyelitis. BMC Musculoskelet Disord. 2024;25(1):474. Published 2024 Jun 17. doi:10.1186/s12891-024-07576-x
18. Cody EA, Scott DJ, Easley ME. Total ankle arthroplasty: a critical analysis review. JBJS Rev. 2018;6:e8.
19. Abar B, Kwon N, Allen NB, et al. Outcomes of surgical reconstruction using custom 3D-printed porous titanium implants for critical-sized bone defects of the foot and ankle. Foot Ankle Int. 2022;43(6):750-761. doi:10.1177/10711007221077113
20. D'Amelio A, Van Lieshout EMM, Wakker AM, Verhofstad MHJ, Van Vledder MG. 3D-printed patient specific instruments for corrective osteotomies of the lower extremity. Injury. 2022;53 Suppl 3:S53-S58. doi:10.1016/j.injury.2022.08.069
21. Dekker TJ, Steele JR, Federer AE, Hamid KS, Adams SB Jr. Use of patient-specific 3D-printed titanium implants for complex foot and ankle limb salvage, deformity correction, and arthrodesis procedures. Foot Ankle Int. 2018 Aug;39(8):916-921.
22. Yang X, Liu P, Zhang Y, Lu J, Zhao H. Bioprinting-Enabled Biomaterials: A Cutting-Edge Strategy for Future Osteoarthritis Therapy. Int J Nanomedicine. 2023;18:6213-6232. Published 2023 Nov 1. doi:10.2147/IJN.S432468
23. Ricles LM, Coburn JC, Di Prima M, Oh SS. Regulating 3D-printed medical products. Sci Transl Med. 2018;10(461):6521