ADVERTISEMENT
Decoding the Molecular Blueprint of Therapeutics in Dermatology
© 2024 HMP Global. All Rights Reserved.
Any views and opinions expressed are those of the author(s) and/or participants and do not necessarily reflect the views, policy, or position of The Dermatologist or HMP Global, their employees, and affiliates.
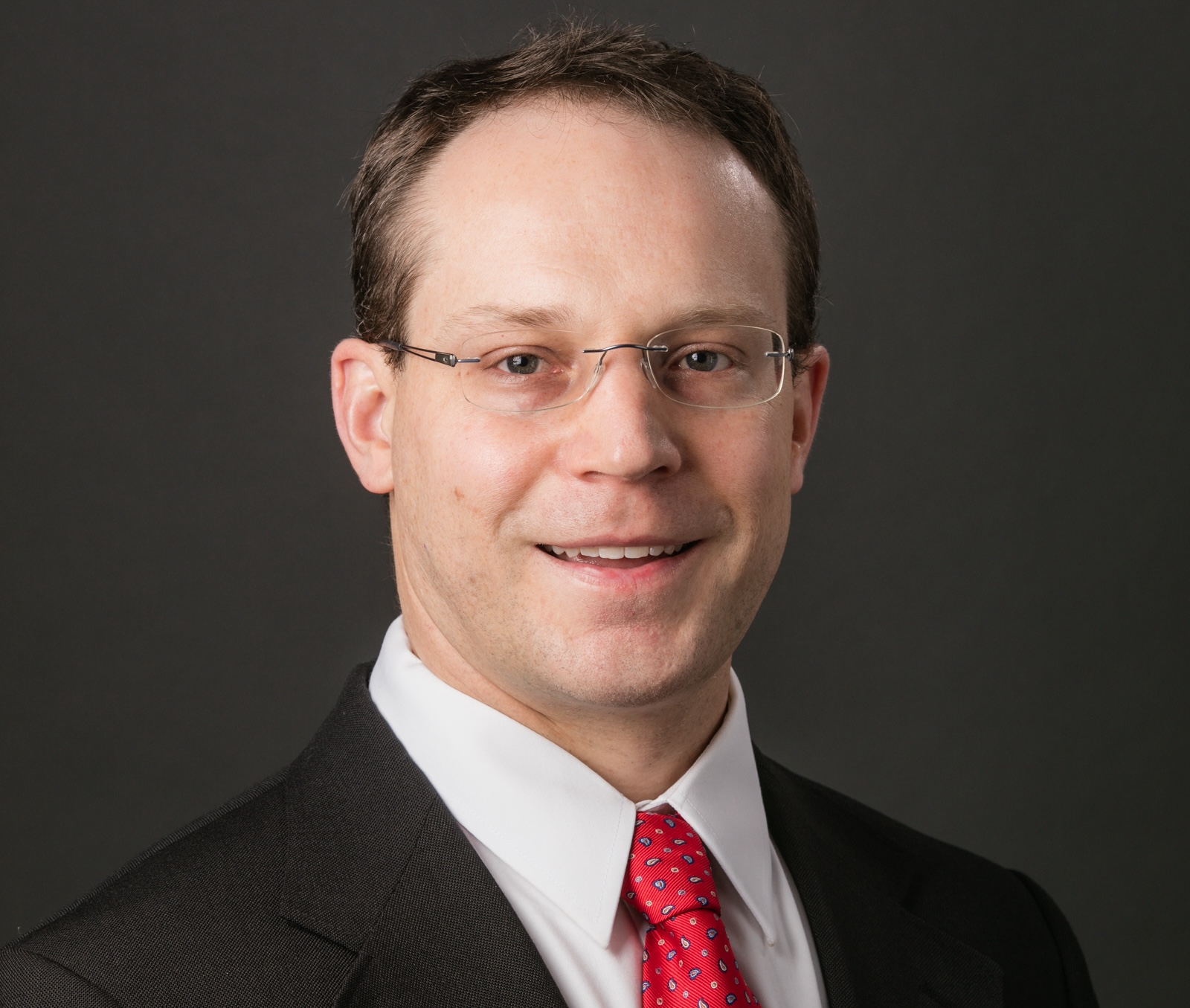
There is an old saying that structure dictates function; if you do not understand the structure, you cannot fully appreciate the function. Crystallography is a key scientific method to unlock the secret/hidden workings of biologic systems. It is akin to a mechanical clock; you may see the face of the clock, but it is all the gears and pieces that create the time-keeping that empower its function. Similarly, crystallography allows us to look deeper into macromolecules, such as proteins or nucleic acids, at an atomic resolution level to determine, analyze, and see their structure. When we think about macromolecules in the body, it is one thing to generally know what they do, but to understand how they work at the level of atoms and bonds generates knowledge that can translate science into therapies and better patient care.
Intermediate Filaments
When you think of intermediate filaments, you might think of hair, nails, and skin because you can see those. But there are also filaments within the cells of the body that are signaling and driving important cellular processes. There are 3 main cytoskeleton systems in cells: intermediate filaments, actin microfilaments, and microtubules. The importance of studying intermediate filaments stems from their relationship to numerous diseases in which there are abnormalities (i.e., mutations or variants) in intermediate filament proteins or from pathogenic roles in cancer. Understanding the fundamental structure-function relationship of intermediate filaments is essential to help understand the disease pathogeneses related to them. We already have therapies that target actin microfilaments and microtubules for cancer and other conditions, including actinic keratosis, but there are no US Food and Drug Administration (FDA)-approved therapies targeting an intermediate filament. Our laboratory is trying to change that.
Skin barrier proteins are a primary area of focus in our laboratory because of their role in many human diseases and, despite decades of study, many remain poorly understood. In the beginning, we focused on understanding the molecular underpinnings of the human skin barrier. What was the structure of different barrier proteins and how might they interact with each other? This is a clinically relevant question for atopic dermatitis (AD) and other disorders where compromise of the skin barrier predisposes to inflammation, scaling, infection, contact dermatitis, and/or itch. We determined crystal structures of 2 important barrier proteins: filaggrin,1 which stands for filament aggregating protein, and keratin 1/10 complex.2
After the initial keratin 1/10 structure, our laboratory investigated intermediate filament assembly mechanisms with the hypothesis that this knowledge could lead to therapeutic innovation. One mature keratin filament, for example, may contain 40 individual keratin proteins, making knowledge of how they come together essential. Moreover, the structural and functional differences between types of filaments, such as keratins vs vimentin, remain understudied. Recently, we published a study in EMBO where we identified a new structural mechanism regulating how intermediate filaments first assemble at the tetramer step, on the way to forming mature, large, multi-subunit assemblies.3 From this discovery, we are developing drug therapies to target and disrupt different intermediate filament systems in hopes of treating their associated diseases.
Species-Specific Mechanism of Action
Our laboratory is focused on unraveling the molecular mechaisms behind acne pathogenesis and treatment, particularly through the lens of tetracycline-class oral antibiotics, which have been a mainstay in acne therapy. In this context, we explored the narrow-spectrum antibiotic sarecycline to understand its unique molecular interactions with Cutibacterium acnes (C. acnes), the bacterium implicated in acne. Narrow-spectrum is important for patients because it potentially offers less disruption of the gastrointestinal microbiome and less risk of antibiotic resistance, among other benefits. Our investigation aimed to discern how this narrow-spectrum antibiotic's mechanism of action differs from traditional broad-spectrum tetracyclines, such as doxycycline and minocycline, at a molecular level, especially in terms of its structure-function relationship and its ability to modulate inflammation and bacterial proliferation in acne lesions.
Delving into the molecular architecture, we discovered that sarecycline exhibits a unique interaction with the bacterial ribosome. Ribosomes work by decoding messenger RNA (mRNA) and translating it into a protein. We discovered that one of the chemical moieties on sarecycline, the carbon 7 extension unique to that molecule, extends into the mRNA channel of the ribosome and interacts with mRNA—a mechanism not observed with other tetracyclines. This insight was gleaned from crystallographic analysis of the ribosome from the organism Thermus thermophilus, serving as a proxy to understand the drug’s interaction with the clinically relevant C. acnes ribosome.4 Interestingly, clinicians have used antibiotics to treat acne for over 50 years but without knowing what the actual structure of the clinically relevant target was. Our laboratory addressed this gap.
Highlighting the importance of species-specific research, our findings underscore that even subtle differences in the molecular structures of pathogens can significantly impact the therapeutic profile of drugs. Our pioneering work in cryogenic electron microscopy determined the first C. acnes ribosome structure, revealing a previously unidentified second binding site for sarecycline in the exit tunnel where the protein is being synthesized and exits the ribosome.5 This work emphasizes the role of structural biology in advancing dermatologic treatment and broadening our understanding of antibiotic mechanisms for the ultimate benefit of both clinicians and patients.
Structure-Based Drug Design
The hope is that the structures we uncover serve as springboards to medication innovation, a concept known as structure-based drug design. With the advent of artificial intelligence (AI), we are seeing the pairing of AI’s machine power with structure-based drug design. We are entering an era where AI rapidly does a superior job of exploring millions of chemicals and how they interact with a target, accelerating the steps of drug discovery and shortening the time to new therapies. Maybe this technology can foster newer treatments for rare diseases or reduce cost barriers to patients. One of the mottos of our laboratory is that you cannot really understand how a disease process works or how a drug works unless you understand its related atomic resolution structure(s). We strive to know the details of the full structure-function relationship, an outcome we termed the “structurotype” because it complements the genotype and phenotype correlation in health and disease.6 Partnering earlier with industry in pipeline development is advantageous. Many drugs are developed where we have a general understanding of how they work, we have preclinical data, and then clinical trial outcomes. Drugs often get approved and are being prescribed to patients without necessarily having full elucidation of their molecular mechanism of action. We are in an era of precision, genomics-driven medicine, tailoring medicine to specific disease endotypes that patients have. It is difficult to comprehend how one can fully care for the dermatology patient if both the provider and the patient do not actually understand how the medications are working. I believe to better serve our patients, we must know how our drugs are working, not just at a superficial level, but at a substantial molecular level. The academic-industry partnership is critical to advancing patient care, innovating therapies, and elucidating mechanisms and function, while ensuring drug efficacy and patient safety.
Numerous drugs are being approved for the same dermatologic disease, creating competition in these disease spaces, whether it is psoriasis, AD, acne, or hidradenitis suppurativa (HS). What clinicians and patients need is differentiation based on science. What is unique about these molecules? How do I know what to use for which patient? For example, as seen in our recent publication in JID Innovations,7 we took the IL-23 biologics for psoriasis and asked the question: What is the molecular difference between them? How is it that they bind to IL-23 differently than each other? Why has risankizumab demonstrated the highest efficacy among IL-23 biologics? We are excited about this work because we can show that there are unique structural features where these anti-IL-23 antibodies are binding, which is called the epitope. We found that there are distinct chemical and structural differences in the p19 epitopes of the 3 p19-specific IL-23 biologics. With these drugs, we can now correlate their epitope’s structural properties with Psoriasis Area and Severity Index 90 and 100 responses in patients. To be able to truly have the structure-function and clinical response correlation is fascinating, and we are interested in understanding more about how the structural nuances influence drug efficacy and disease response. These goals have spear-headed additional work on phosphodiesterase-IV inhibitors, antimicrobials, targeted biologics, Janus kinase (JAK) inhibitors, and more.
Master Key of Dermatology
The advent of oral JAK inhibitors has markedly advanced the standard of care for patients with AD, introducing a paradigm shift in its therapeutic management. These agents, by virtue of their novel mechanism that entails the inhibition of multiple cytokines involved in the disease pathophysiology through the JAK-STAT signaling pathway, have facilitated a targeted and efficacious approach to treatment. Notably, upadacitinib and abrocitinib have been instrumental in enabling many patients to achieve complete disease control (itch resolution and skin clearance) within a comparatively short time frame, an outcome not as easily achievable with conventional therapies and injectable biologics. Moreover, the long-term safety profiles of upadacitinib and abrocitinib have provided both patients and clinicians with a high degree of reassurance, mitigating concerns associated with the chronic administration of systemic therapies.
The untapped potential of oral JAK inhibitors extends beyond AD, promising a broader therapeutic horizon across various immune-mediated skin disorders. The mechanistic versatility of JAK inhibitors, capable of modulating multiple immune pathways, positions these agents as potentially transformative in the management of a spectrum of dermatologic conditions characterized by dysregulated immune responses. Preliminary investigations and clinical trials are beginning to unveil the efficacy of JAK inhibitors in conditions such as HS, vitiligo, and alopecia areata, among others, suggesting a burgeoning role for these medicines in dermatology. Given this, it is essential to elucidate the molecular science behind JAK inhibitor function. Our laboratory is investigating the correlation between JAK inhibitor structure and kinase selectivity, how the phosphorylation signal is mechanistically transferred from JAK to STAT efficiently and effectively, and structure-function correlations with clinical efficacy and patient safety.8
The Patient Connection
The traditional bench-to-bedside model, where scientific discoveries move from the laboratory to clinical application, is evolving. Direct patient care offers invaluable insights into unmet needs and patient suffering, guiding scientists to ask more relevant questions. When you are caring for patients face to face, it gives you a different perspective. You hear from the patients themselves, “Here is what bothers me. Here is how I am suffering. Here is what I wish I had.” I think those are powerfully motivating questions for scientists to say, let me now go back to the laboratory and take what I have seen and heard clinically and work to find answers to these questions. This clinic-to-bench-and-back approach not only fosters the development of new therapies but also ensures that research is patient centered. As both a scientist and a clinician, the daily interactions with patients and colleagues fuel my quest for practical solutions, driving the creation of therapies that can truly benefit patients. This synergy between clinical experience and scientific inquiry is essential for meaningful advancements in health care.
References
1. Bunick CG, Presland RB, Lawrence OT, Pearton DJ, Milstone LM, Steitz TA. Crystal structure of human profilaggrin S100 domain and identification of target proteins annexin II, stratifin, and HSP27. J Invest Dermatol. 2015;135(7):1801-1809. doi:10.1038/jid.2015.102
2. Bunick CG, Milstone LM. The X-ray crystal structure of the keratin 1-keratin 10 helix 2B heterodimer reveals molecular surface properties and biochemical insights into human skin disease. J Invest Dermatol. 2017;137(1):142-150. doi:10.1016/j.jid.2016.08.018
3. Eldirany SA, Ho M, Hinbest AJ, Lomakin IB, Bunick CG. Human keratin 1/10-1B tetramer structures reveal a knob-pocket mechanism in intermediate filament assembly. EMBO J. 2019;38(11):e100741. doi:10.15252/embj.2018100741
4. Batool Z, Lomakin IB, Polikanov YS, Bunick CG. Sarecycline interferes with tRNA accommodation and tethers mRNA to the 70S ribosome. Proc Natl Acad Sci U S A. 2020;117(34):20530-20537. doi:10.1073/pnas.2008671117
5. Lomakin IB, Devarkar SC, Patel S, Grada A, Bunick CG. Sarecycline inhibits protein translation in Cutibacterium acnes 70S ribosome using a two-site mechanism. Nucleic Acids Res. 2023;51(6):2915-2930. doi:10.1093/nar/gkad103
6. Wu TT, Eldirany SA, Bunick CG, Teng JMC. Genotype-structurotype-phenotype correlations in patients with pachyonychia congenita. J Invest Dermatol. 2021;141(12):2876-2884.e4. doi:10.1016/j.jid.2021.03.035
7. Daniele SG, Eldirany SA, Damiani G, Ho M, Bunick CG. Structural basis for differential p19 targeting by anti-IL-23 biologics: correlations with short- and long-term clinical efficacy in psoriasis. JID Innov. Published online January 19, 2024. doi:10.1016/j.xjidi.2024.100261
8. Wang J, Batista VS, Bunick CG. Triple-action inhibitory mechanism of allosteric TYK2-specific inhibitors. bioRxiv [Preprint]. 2023:2023.10.09.561507. doi:10.1101/2023.10.09.561507
9. Teng JMC, Bunick CG, Guenthner S, et al. The CONTROL study: a randomized, double-blind vehicle-controlled phase 2b study of novel topical isotretinoin formulation demonstrates improvement in recessive X-linked and autosomal recessive lamellar congenital ichthyosis. J Am Acad Dermatol. 2022;87(6):1455-1458. doi:10.1016/j.jaad.2022.07.028